Authorship
Gucker, Corey; Shaw, Nancy
Publication Date
February 2018
Update
February 2024
Nomenclature
Balsamorhiza sagittata (Pursh) Nutt., hereafter referred to as arrowleaf balsamroot, belongs to the Artorhiza subgenus (Sharp 1935), Heliantheae tribe, and Engelmaniinae subtribe of the Asteraceae family (Cronquist et al. 1994; Moore and Bohs 2003; Weber 2006).
Family
Asteraceae – Aster family
Genus
Balsamorhiza
Species
sagittata
NRCS Plant Code
BASA3 (USDA NRCS 2024).
Subtaxa
No varieties or subspecies are currently recognized by the Flora of North America (Weber 2006).
Synonyms
Balsamorhiza helianthoides (Nutt.) Nutt., Espeletia helianthoides Nutt., and E. sagittata (Pursh) Nutt. (Weber 2006).
Common Names
Arrowleaf balsamroot, breadroot, graydock, spring sunflower (USDA FS 1937; USDA NRCS 2024).
Chromosome Number
Chromosome number is 2n = 38 (Weber 2006).
Hybridization
Hybridization is common within the Balsamorhiza genus where distributions overlap, and the genus is described as “notorious for lack of genetic barriers to hybridization” (Welsh et al. 2015). Intermediate forms resulting from hybridization with Hooker’s balsamroot (B. hookeri), Carey’s balsamroot (B. careyana), hoary balsamroot (B. incana), and rosy balsamroot (B. rosea) have been reported (Ownbey and Weber 1943; Welsh et al. 2015; Taylor 1992; Weber 2006), but these may not represent all potential hybrids. Arrowleaf balsamroot does not hybridize with cutleaf balsamroot (B. macrophylla), which is a highly polyploid species (Weber 2006).
Although common, hybrids may be highly restricted geographically. Arrowleaf balsamroot × Hooker’s balsamroot hybrids are restricted to the ecotones between parent habitats in sagebrush communities, which are deep soils for arrowleaf balsamroot and shallow soils lacking defined horizons for Hooker’s balsamroot (Ownbey and Weber 1943). Research conducted by the Great Basin Native Plant Project found the putative arrowleaf balsamroot × Hooker’s balsamroot hybrids are common in central and northern Nevada and southwestern Idaho (Kilkenny et al. 2023).
A similar type of restricted geographic occurrence for parents and hybrids was reported for overlapping distributions of arrowleaf balsamroot and hoary balsamroot in Wallowa County, Oregon (Ownbey and Weber 1943). Arrowleaf balsamroot occupied fertile mounds, and hoary balsamroot occupied adjacent rocky subsoils. Plants looking like arrowleaf balsamroot and growing in rocky subsoils displayed some hoary balsamroot characteristics. Plants growing on more fertile mounded sites displayed arrowleaf balsamroot characteristics (Ownbey and Weber 1943).
Recognized hybrids include: B. × tomentosa: hoary balsamroot × arrowleaf balsamroot and B. × bonseri: rosy balsamroot × arrowleaf balsamroot (Weber 2006; Hitchcock and Cronquist 2018).
Distribution
Arrowleaf balsamroot is primarily a western species, occurring from the Cascade and Sierra Nevada mountain ranges to the Black Hills of South Dakota. It is found in the Canadian provinces of Alberta, British Columbia, and Saskatchewan and in the U.S. states of Arizona, California, Colorado, Idaho, Montana, Nevada, Oregon, South Dakota, Utah, and Wyoming (USDA FS 1937; Weber 2006). It is widespread at low to mid-elevations east of the Cascades, from southern British Columbia to California, and east to the Rocky Mountains (Hitchcock and Cronquist 2018).
Habitat And Plant Associations
Arrowleaf balsamroot occurs most commonly in grassland (Fig. 1), sagebrush (Artemisia spp.), oak (Quercus spp.) brush, mountain brush, pinyon–juniper (Pinus–Juniperus spp.), ponderosa pine (P. ponderosa), Douglas–fir (Pseudotsuga menziesii), and quaking aspen (Populus tremuloides) communities throughout its range (Kuchler 1964; Welsh et al. 2015; Shiflet 1994; Weber 2006). Although found at sites receiving between 9 and 25 in (229–635 mm) of precipitation, populations are often more dense and plants larger as annual precipitation increases beyond 12 in (305 mm) (Wasser 1982; USDA NRCS 2000; Tilley et al. 2012).
Grasslands. Arrowleaf balsamroot is a common component of fescue (Festuca altaica and F. idahoensis)– and bluebunch wheatgrass (Pseudoroegneria spicata)-dominated rangeland cover types (Shiflet 1994) where typically less than 40% of annual precipitation falls during the growing season (Garrison et al. 1977). In the Blue Mountains of Idaho, Oregon, and Washington, the Idaho fescue (F. idahoensis)-bluebunch wheatgrass-arrowleaf balsamroot vegetation type is common on warm, moist sites, and the bluebunch wheatgrass-Sandberg bluegrass (Poa secunda)–arrowleaf balsamroot vegetation type is common on hot, dry sites (Powell et al. 2007).
Figure 1. Arrowleaf balsamroot growing in a Wyoming grassland. Photo: USDI BLM WY 404 SOS.
Shrublands. Arrowleaf balsamroot is most common in upland rangeland shrubland types dominated by antelope bitterbrush (Purshia tridentata), basin big sagebrush (A. tridentata subsp. tridentata), Wyoming big sagebrush (A. t. subsp. wyomingensis), and mountain big sagebrush (A. t. subsp. vaseyana) (Shiflet 1994). It can also be found in low sagebrush (A. arbuscula) communities (Shiflet 1994), but here it is likely restricted to deep soil microsites (S. Monsen, USFS retired, personal communication, March 2018). Arrowleaf balsamroot abundance is typically greatest in mesic sagebrush sites at high elevations or north–facing slopes (Young and Evans 1979). At Craters of the Moon National Monument, south-central Idaho, it occurred on less favorable, rocky outcrops in mountain big sagebrush/bluebunch wheatgrass communities (Day and Wright 1985).
Mountain brush sites with Gambel oak (Quercus gambelii), mountain mahogany (Cercocarpus spp.), snowberry (Symphoricarpos spp.), or serviceberry (Amelanchier spp.) are also common arrowleaf balsamroot habitats (Brown 1982). In the Teton Range of eastern Idaho, arrowleaf balsamroot occurred in all 15 Saskatoon serviceberry (A. alnifolia) stands sampled. These stands occurred on south to southwest slopes with young rocky loam or clay soils at elevations of 6,500 to 7,150 ft (1,980–2,180 m) (Major and Rejmanek 1992). In the south Warner Mountains of Modoc County, California, the curl-leaf mountain mahogany (C. ledifolius)/arrowleaf balsamroot habitat type occupies steep, dry, southeastern slopes (Riegel et al. 1990).
Woodlands. In open, low– to mid–elevation conifer (Fig. 2) and quaking aspen woodlands, arrowleaf balsamroot can be abundant. It is a characteristic species of western juniper (J. occidentalis)/big sagebrush/bluebunch wheatgrass rangeland types (Shiflet 1994). On Steens Mountain of southeastern Oregon, it was positively associated with increasing soil moisture in western juniper encroached vegetation on south–facing slopes when stands across a heterogeneous landscape were evaluated (Peterson and Stringham 2009).
Figure 2. Arrowleaf balsamroot growing in mixed–conifer forest openings in central Idaho. Photo: N. Shaw, USFS-retired.
Arrowleaf balsamroot was also very common in ponderosa pine/bluebunch wheatgrass, Idaho fescue, and antelope bitterbrush communities in Oregon and Washington, but in these communities in south–central Oregon, arrowleaf balsamroot was restricted to residual soils and absent from pumice soils (Franklin and Dyrness 1973).
Elevation
Arrowleaf balsamroot occupies sites ranging from 330 to 9,850 ft (100–3,000 m) in elevation but is most common between 2,950 and 8,200 ft (900-2,500 m) (Stevens and Monsen 2004; Weber 2006). The elevational range reported for arrowleaf balsamroot in Utah is 4,400 to 9,900 ft (1,340–3,020 m) (Welsh et al. 2015) and for California it is 4,600 to 8,530 ft (1,400–2,600 m) (Hickman 1993).
Soils
Arrowleaf balsamroot occurs on sites with deep, well–drained, generally neutral, fine- to coarse-textured soils. While plants can withstand periodic saturation, a shallow water table is not tolerated (Tisdale 1947; Taylor 1992; USDA NRCS 2000; Stevens and Monsen 2004). Plants do best in soils that are only weakly acidic or alkaline (pH 6.5–8) and have low salinity (Wasser 1982; Shaw and Monsen 1983; Tilley et al. 2012; Eldredge et al. 2013).
Arrowleaf balsamroot was a dominant forb in climax grasslands in eastern Idaho on loamy soils 8 to 16 in (20–41 cm) deep (Rumsey 1971). In a survey of sagebrush–grasslands in northern Utah, northeastern Nevada, southern Idaho, and west–central Wyoming, arrowleaf balsamroot was generally absent from shallow soils unless porous or fractured rock allowed for water accumulation and deeper root growth. Plants were also common on Aridisols and Mollisols but often absent from deeply freezing soils without snow insulation or with natric and argillic horizons (Passey et al. 1982).
When 23 mountain grassland stands above about 4,000 ft (1,200 m) in central Idaho were compared, arrowleaf balsamroot was associated with high clay content soils from basalt parent materials (Mueggler and Harris 1969). In high–elevation grasslands of southeastern Washington, plants were most common on dry slopes where soils were 25% sand, 73.5% silt, and 1.5% clay (Weaver 1915). In the Bear River Range of northeastern Utah and southeastern Idaho, plants were almost entirely restricted to dolomite soils when dolomite and quartzite soils were compared. Soil pH, silt content, and percent moisture were significantly greater (P < 0.01) and sand content was significantly lower for dolomite than for quartzite soils. Dolomite soils had an average pH of 7 (range: 6.2–8.3) and averaged 44% sand, 42% silt, and 14% loam (Neely and Barkworth 1984).
Description
Arrowleaf balsamroot is a large, leafy, long–lived perennial with striking early season yellow flowers. Its root system and the large, caudex with multiple growing points, give the plant its drought tolerance and ability to recover vegetatively following top–kill (Welsh et al. 2015; Kitchen 1994; Hitchcock and Cronquist 2018). The large, thick, partially woody taproot contains a balsam–scented resin and is the reason for the genus name (USDA FS 1937).
Below ground. Roots of 25 excavated plants in the Palouse Prairie of southeastern Washington extended 5.5 to 9 ft (1.7–2.7 m) deep and spread 3 ft (1 m) laterally via many horizontal branches (Weaver 1915, 1958). The upper, older portion of the taproot was covered with deeply furrowed bark. Maximum taproot diameter was 4 in (10 cm). Numerous lateral roots appeared about 6 in (15 cm) below the soil surface. These measured more than 1 in (2.5 cm) in diameter and ran horizontally for up to 2 ft (0.6 m) before turning downward to depths of 5 ft (1.5 m) (Weaver 1915).
Above ground. Plants are strongly tufted, densely leafy from the base, and large (up to 2.5 ft [75 cm] tall when flowering) (Fig 3; Blaisdell 1958; Welsh et al. 2015). Weaver (1915) counted up to 39 individual shoots arising from a single crown measuring 9 in (23 cm) in diameter. Stems are almost leafless and end in large, yellow, typically solitary, sunflower-like inflorescences (Weber 2006; Pavek et al. 2012; LBJWC 2017). Stems and basal leaves are covered in dense, soft hairs giving them a gray–green color (Hitchcock and Cronquist 2018; Weber 2006). Young leaves are silver with fine, felt-like hairs, especially on the undersides, but leaves become less hairy and greener with age (Hitchcock and Cronquist 2018). Basal leaves are arrowhead to heart-shaped and up to 18 in (46 cm) long and 6 in (15 cm) wide with long petioles. Stem leaves, if present, are few, reduced, and almost bract-like (Welsh et al. 2015; Taylor 1992; Cronquist et al. 1994).
Figure 3. Arrowleaf balsamroot growing in Wyoming. Photo: USDI BLM WY 050 SOS.
Flower heads are terminal and generally solitary, but can number as many as three (Fig. 4). They are 2.5 to 4 in (6.3–10 cm) wide and are comprised of outer, yellow, female ray flowers and inner, yellow, perfect disk flowers (USDA FS 1937; Welsh et al. 2015; Taylor 1992; Weber 2006; LBJWC 2017; Hitchcock and Cronquist 2018). The number of ray flowers ranges from 8 to 25 but is most commonly between 13 and 21 (Stubbendieck et al. 1986; Cronquist et al. 1994). Both ray and disk flowers are fertile and produce seed, although the greatest percentage of seed is produced by disk flowers (Monsen, USFS [retired], personal communication, March 2018). Fruits are smooth, three- to four-angled cypselas up to 0.75 in (2 cm) long and 2 mm wide (Wasser 1982; Belcher 1985; Welsh et al. 2015; Hickman 1993). Cypselas are brown to black when mature and lack a pappus or bristles (USDA FS 1937; Belcher 1985). Arrowleaf balsamroot cypselas, although not truly achenes, are widely referred to as such. Throughout this review, seed refers to the fruit or cypsela and the seed it contains. This is because the seed remains with the fruit during cleaning and it is the fruit that is planted.
Figure 4. Arrowleaf balsamroot flowering is fairly uniform. Photo: USDI BLM WY040 SOS.
Plant size, seed size, and reproductive output can vary annually and by site. At the USDA ARS Sheep Experiment Station (USSES) near Dubois, Idaho, leaf height averaged 5.8 to 11.4 in (14.8–28.9 cm) over a 19–year period (Blaisdell 1958). Flower stalk heights ranged from 0 to 17.8 in (44.6 cm), and flower stalk number ranged from 0 to 12.8 over a 9–year period. Increased early growth was associated with high temperatures, low precipitation, and relatively clear skies (Blaisdell 1958). In a drought year with winter precipitation 1.5 in [3.8 cm] below average, plants produced just 60% of the height attained in normal precipitation years at the USSES. Plants produced some flowers but little seed (Pechanec et al. 1937). In a single year of observations, plants at a high–elevation site (5,988 ft [1,825 m]) were smaller and generally produced fewer and lighter seeds than plants at lower elevations (3,609 to 5,003 ft [1,100–1,525 m]) (Amsberry and Maron 2006).
Reproduction
Arrowleaf balsamroot reproduces sexually from seed. Seeds are large, without dispersal appendages, and fall close to parent plants (Fig. 5). Although seeds are highly dormant when ripe, the seed bank is not persistent (Kitchen 1994; Amsberry and Maron 2006). Seed production is considered more reliable at high–moisture, high–elevation sites than at hot, dry, low–elevation sites (Stevens and Monsen 2004). Regardless of site conditions, predation by insects and grazing by mammals and late frosts often limit seed production, and the lack of soil–stored seed is largely attributable to rodent predation, which is typically relentless (Stevens and Monsen 2004; Amsberry and Maron 2006; Pearson and Callaway 2008).
Reproductive maturity is delayed for this long–lived species. Under the best growing conditions, plants require 3 to 4 years to produce seed. However, it can take 10 or more years to produce seed under less ideal growing conditions (Stevens and Monsen 2004). Reproductive output can be cyclical, with mast seed years and years with minimal or no seed production resulting from late–spring frosts or loss of seed crops from herbivory. Mast seed years can often be linked to favorable patterns and abundance of precipitation and a lack of late–spring frosts (Kitchen 1994; S. Monsen, USFS retired, personal communication, March 2018). Monsen’s observations also suggest that seed production is often lower following site disturbance.
Arrowleaf balsamroot is described as a long–lived species, but only one study attempted to estimate its life span and survival rate (Treshow and Harper 1974). Life span was estimated at 40 years or more when researchers evaluated taproot growth rings, annual mortality, and annual vegetative replacement and survival rates. Over four growing seasons, annual mortality averaged 3% (range: 1–5%). Annual vegetative replacement rate averaged 4%, and survival rate averaged 97% (Treshow and Harper 1974).
Phenology
Arrowleaf balsamroot is an early season species (Blaisdell et al. 1952; Kitchen 1994). Flowers are generally produced in the spring and fruits in early summer, but site and weather patterns impact phenology. At the USSES, plants began growing about 20 days after an area was free of snow (around April 20). Flowering was complete in very early June. Seed was ripe by mid–June and dispersed about a week later (Blaisdell et al. 1952). When phenology was monitored from 1928 to 1937 on multiple sites east of the Continental Divide in Montana and in Yellowstone National Park, growth began between April 3 and May 13, flowering started between April 27 and June 18, flowering ended between May 15 and July 8, and fruits were ripe between June 13 and August 2 (Schmidt and Lotan 1980).
Seed ripening depends on elevation and moisture and occurs later on more moist and/or higher elevation sites (USDA FS 1937). In western Montana, seed maturation at a 3,609–ft (1,100 m) site was 3 weeks earlier than at a 5,988–ft (1,825 m) site (Amsberry and Maron 2006). In a drought year when winter precipitation was 1.5 in (3.8 cm) below average, plants began growing on March 25, about 1 month before usual, in sagebrush–bunchgrass communities at USSES. Plants were dry by May 1 and produced little seed (Pechanec et al. 1937).
Figure 5. Arrowleaf balsamroot seed head and seeds. Photo: USDI BLM OR030 SOS.
Comparisons of arrowleaf balsamroot phenology made over time in Grand Teton National Park suggest the species is emerging and flowering earlier than in the past. When phenology records from 1974 to 1979 and 1988 were compared to those made from 2016 to 2018, arrowleaf balsamroot emerged about 21 days earlier in the 2016 to 2018 time period than in the 1970s (Bloom et al. 2018).
Arrowleaf balsamroot flowered earlier, longer, and produced more seedheads with experimental warming and snow removal at field sites in Grand Teton National Park (Durney et al. 2022). Researchers also found that the effects of heating and snow removal on flowering onset and maximum floral display increased over time. The study was conducted in a montane meadow (a mix of sagebrush, grasses, forbs with 50% bare ground at 6,890 ft [2,100 m]) from 2013 to 2019. It included four treatments: decreased spring snowpack through snow removal; warming by passive heating using a structure with a plastic top that allowed precipitation through; warming and snow removal combined; and a control. Snow removal treatments resulted in the removal of 22 to 32 in (55-80 cm) of snow in years of deep accumulations and warming treatments increased the temperature of plant and soil surfaces by 36 °F (2 °C). The onset of flowering was advanced 4 days with snow removal alone, 3.8 days with removal and warming, and 1.9 days by heating alone compared to controls. Duration of flowering was extended by 5 days with warming and snow removal, 3.6 days with snow removal only, and 3.4 days with warming alone compared to controls. Maximum floral display was 1.2 flowers greater in warming and snow removal treatments than controls. Plants in warming and snow removal plots produced the most flowers and in controls the least, regardless of the year. Plants that began flowering earlier also bloomed for a longer period of time. Because flowering onset occurred earlier under snow removal than for warming treatments, researchers concluded that snow removal drove the trend, suggesting that earlier access to light enables photosynthesis, fostering earlier growth and onset of flowering for arrowleaf balsamroot (Durney et al. 2022).
Breeding System
Arrowleaf balsamroot flowers can be insect– or wind-pollinated (Stevens et al. 1996), cross pollination is key to seed production (Cane 2005). When 25 plants from two populations near Logan, Utah, were studied, manually self–pollinated flowers produced 18 plump seeds/flower head, whereas manually out-crossed flowers produced 30 plump achenes/flower head. Flowers protected from pollinators produced almost no achenes (Cane 2005).
Pollination
Native bees are important pollinators (Cane 2005). Based on observations made in 30 arrowleaf balsamroot populations in 5 states, multiple genera of mostly non-social bees (Anrena, Dialictus, Halictus, and Osmia spp.) visited arrowleaf balsamroot flowers (Cane 2011). In pollinator surveys conducted in Utah, researchers collected 797 bees from 5,513 plants at 41 mostly sagebrush steppe sites. The most frequently collected bees were mason bees (Osmia spp.), mining bees (Andrena spp.), long–horned bees (Eucera spp.), furrow bees (Halictus spp.), sweat bees (Laioglossum spp.), and cuckoo bees (Nomada spp.). Total bee visitors represented 15 different genera and 32 unique species (Cane and Love 2016).
Ecology
Arrowleaf balsamroot is a mid– or late-seral species in grasslands and shrublands, and an early– to mid–seral species in woodlands where it becomes less abundant or disappears with increased shading. Following disturbances in communities where it is already established, arrowleaf balsamroot generally recovers quickly and is present soon after disturbance. However, in suitable habitats where it was not present before a disturbance, it is not an early colonizer.
Arrowleaf balsamroot was reported in many climax and near climax grasslands and shrublands across its range (Tisdale 1947; Rumsey 1971; Franklin and Dyrness 1973). While some grazing of these communities, led to increased arrowleaf balsamroot abundance, heavy grazing often resulted in decreased abundance (Tisdale 1947; Johnson and Swanson 2005).
In general, plants do not tolerate dense shade and grow in sun to partial shade conditions (McConnell and Smith 1970; Habeck 1976; Gruell et al. 1982). In a restored site in south–central Idaho, presence and importance of arrowleaf balsamroot increased with increased distance from antelope bitterbrush shrubs, and plants grew better in open than shaded conditions (Monsen and Shaw 1983). In western Montana, plant abundance was reduced dramatically or completely in ponderosa pine forests as the density of young conifers increased and reduced the abundance and size of openings between mature trees (Gruell et al. 1982). In ponderosa pine and Douglas–fir forests in the Selway–Bitterroot Wilderness, arrowleaf balsamroot was most common on warm aspects with low tree cover and duff depths (< 1 cm). As tree cover and duff depths increased, arrowleaf balsamroot decreased in abundance or disappeared (Habeck 1976). In the Blue Mountains of northeastern Oregon, frequency of arrowleaf balsamroot was 15% in untreated forests with the greatest tree densities and 34% in thinned and burned plots with the lowest tree densities (Metlen et al. 2004).
Seed And Seedling Ecology
Arrowleaf balsamroot seed, although dormant, likely to prevent same-season germination, does not persist long in the soil seed bank (Kitchen 1994; Amsberry and Maron 2006; Crone et al. 2009). However in revegetation monitoring, Stevens and Monsen (2004) found that seedlings would emerge for 2 to 3 years after fall seeding. In soil samples collected from British Columbia’s Okanagan Valley, just 0.009 arrowleaf balsamroot seed/ft² (0.1/m²) was recovered, where aboveground density of plants was 0.3 plants/ft² (3/m²). Soil was collected in late May or early June, likely before arrowleaf balsamroot seed had matured (Clements et al. 2007).
Studies suggest that seedling recruitment may be improved with increased seed availability, rodent exclusion, and disturbance. In western Montana, seedling recruitment was low when as many as 100 seeds were added to native bunchgrass plots (1.6 ft [0.5] m × 1.6 ft [0.5 m]) lacking adult arrowleaf balsamroot plants (Amsberry and Maron 2006). In plots seeded with 100 seeds, cumulative recruitment averaged just 1.6 seedlings/plot in the first year, and there was no significant relationship between seed density and recruitment for the 5 seed densities tested (0, 13, 25, 50, and 100). In the following year, however, cumulative recruitment was positively influenced by seeding density (P < 0.002), although not at all sites (Amsberry and Maron 2006). A follow-up study in the same area, found that recruitment of arrowleaf balsamroot was significantly (no p value reported) reduced in seed addition plots with rodents than in plots where rodents were excluded. The primary seed consumer in the area was the North American deer mouse (Peromyscus maniculatus) (Maron et al. 2019). In central Idaho Douglas-fir forests where arrowleaf balsamroot is considered a mid-seral understory species, seedling establishment was common following disturbances (fire, logging, mechanical scarification, or some combination) (Steele and Geier-Hayes 1995).
Seed dispersal is necessary for reproduction and colonization of new sites. When abandoned agricultural fields (≤ 52 years since tilling) and adjacent never-tilled vegetation were compared, cover was significantly (P < 0.05) greater in never-tilled vegetation. Cover averaged 15.4% in intact grasslands and 0.1% in old fields, which suggested slow colonization of adjacent sites (Kulmatiski 2006).
While optimal conditions for good seedling recruitment are not clear, a combination of factors including good seed production paired with limited seed predation followed by favorable winter and spring precipitation and temperature patterns may be involved.
Disturbance Ecology
Arrowleaf balsamroot is generally tolerant of disturbance; decreases in abundance, however, are possible following particularly severe or continual disturbances. Plants are most resistant to disturbance in the summer or fall when not actively growing. Mechanical or chemical treatments that impact the root system are generally not tolerated. In Rich County, Utah, arrowleaf balsamroot was an indicator of burned sites when the species composition of chemically, mechanically (disking, aerating, or harrowing), and burned sagebrush sites were compared. Sites were disturbed up to 50 years earlier (Ripplinger et al. 2015).
Fire response. Plants typically survive even severe fire by sprouting from the caudex after burning (Bradley et al. 1992). Increases in abundance following fire are common. In cases where plants are damaged and abundance is reduced by fire, recovery to prefire abundance levels from increased growth of established plants and seedling recruitment on burned sites is typical within 2 to 5 years (Houston et al. 2001; Miller et al. 2013). Fire tolerance may be greatest when plants are dormant (Wasser 1982). However, one study reported that an arrowleaf balsamroot plant was dead following a late August fire in big sagebrush in northern Nevada. Surface temperatures of the fire reached 1,200 °F (650 °C), and soil temperatures reached 250 °F (120 °C) where the plant was killed (Zschaechner 1985).
Increased abundance of arrowleaf balsamroot was common in studies reporting the short-term effects of fire. At mountain big sagebrush sites near Salmon, Idaho, arrowleaf balsamroot increased following a severe spring prescribed fire that killed all sagebrush plants. Abundance increases occurred into the second post-fire year on less severely burned sites and into the third post-fire year on the most severely burned sites (Kuntz 1982). In ponderosa pine-montane grasslands in the Selway-Bitterroot Wilderness of Idaho, production was higher on burned and unburned plots in all 4 post-fire years with peak production on burned plots in the third post-fire year (Merrill et al. 1980). Density of plants increased for 4 years after a July fire in big sagebrush/Thurber’s needlegrass (Achnatherum thurberianum) communities near Reno, Nevada. Density of arrowleaf balsamroot was 0.009 plant/ft² (0.1/m²) at 1 month after fire and 0.03 plant/ft² (0.3/m²) 4 years after fire (Young and Evans 1978). Six years following a July fire, arrowleaf balsamroot cover was similar on burned (low-, moderate-, and high-severity) and unburned sagebrush and western juniper sites in Owyhee County, Idaho. Cover averaged 0.65% on unburned and 0.76% on burned sites (Weiner et al. 2016).
In an extensive study of post-fire recovery in various vegetation types in northeastern Oregon, Johnson (1998) found that arrowleaf balsamroot cover consistently increased, often within the first year, following moderate to severe fires in bluebunch wheatgrass communities. However, cover generally decreased for the first 1 to 5 years following fire in Idaho fescue grasslands. In woody-dominated communities, cover of arrowleaf balsamroot often increased slightly after fire, but following a low-severity fire in a Douglas-fir/white spiraea (Spiraea betulifolia) forest, arrowleaf balsamroot cover increased 25% within 5 years of the fire (Johnson 1998).
Cover of arrowleaf balsamroot was lower although not significantly so following an August fire in bluebunch wheatgrass-Sandberg bluegrass-arrowleaf balsamroot habitats in the Salmon River Canyon in northern Idaho. Cover was 13% before the fire, 6.8% in post-fire year two, and 7.4% in post-fire year three (Gucker and Bunting 2011).
Studies investigating longer term fire responses suggest that periodic fire, especially in woodlands, may encourage arrowleaf balsamroot growth and persistence. In southwestern Idaho’s Owyhee Mountains, response of arrowleaf balsamroot to fire in a western juniper community ranged from unchanged to four times greater by the first or second post-fire year. From these findings, Bunting (1987) predicted that arrowleaf balsamroot would increase if western juniper stands burned at an interval of 25 years or less, remain unchanged if fires occurred every 50 years, and decrease if fires occurred every 100 years. When Koniak (1985) compared 1- to 60-year-old burned singleleaf pinyon-Utah juniper (P. monophylla–J. osteosperma) sites in Nevada and California, arrowleaf balsamroot occurred in all post-fire seral stages, but percent occurrence was significantly greater on 1- to 8-year–old burned plots than on 22- to 60-year-old burned plots (P < 0.05) (Koniak 1985).
Grazing response. Arrowleaf balsamroot is grazed and preferred by many animals (see Wildlife and Livestock Use section). Light to moderate grazing may be tolerated but heavy grazing is not, and plants are more sensitive to spring than fall grazing (Blaisdell and Pechanec 1949; Laycock 1967). Research and observations from the USSES indicate that plant size and flower production are reduced and plants can be killed with heavy sheep grazing (Craddock and Fording 1938). In southwestern Alberta bunchgrass communities, the absence or scarcity of arrowleaf balsamroot distinguishes poor rangeland condition from good or fair condition (Hanson 1951).
Additional studies and observations from the USSES indicate that arrowleaf balsamroot abundance was reduced dramatically with spring sheep grazing (Bork et al. 1998). In pastures where heavy fall grazing from 1924 to 1949 was changed to heavy spring grazing from 1950 to 1963, production decreased by more than 85%. These pastures were in good condition in 1950 and poor condition in 1964. In continually fall-grazed and protected pastures, production increased between 1950 and 1964 (Laycock 1967).
Biomass removal resulted in reductions in the following year’s growth at the USSES (Blaisdell and Pechanec 1949). All plants clipped during the growing season had reduced herbage and flower stalk production the following year. Reductions were greatest when clipping occurred in late May and early June when plants were in full flower or had produced seed (Table 1).
Table 1. Reductions in next season’s growth after clipping arrowleaf balsamroot plants at the USDA ARS Sheep Experiment Station near Dubois, Idaho (Blaisdell and Pechanec 1949).
Clipping date |
4/27 |
5/7 |
5/18 |
5/28 |
6/17 |
6/28 |
Plant phenology |
Initiated growth |
Flower stalks, no blooms |
Flowers, not full bloom |
Full bloom |
Ripe seed |
Drying |
Production (%) of unclipped plants in the following year |
89.7 |
69.6 |
53.0 |
40.1 |
49.3 |
62.5 |
Arrowleaf balsamroot reductions, however, were not reported on every grazed site. At sites near Penticton, British Columbia, frequency was not significantly different on grazed and protected sites in the transition zone between sagebrush and ponderosa pine vegetation (Wikeem and Pitt 1991). Protected sites were ungrazed by large mammals for 22 years. Grazed sites were enclosures used year-round by bighorn sheep (Ovis canadensis) and grazed at 60 to 70% use levels for 2 years. Leaf length, basal diameter, stem length, number of stems, and plant frequency were not different on protected and grazed sites (Wikeem and Pitt 1991). When grazed and mostly protected big sagebrush grasslands were compared in Sweetwater County, Wyoming, frequency of plants was 2% on naturally protected sites and 70% on grazed sites used by domestic sheep in the winter. Sand content was greater and clay content was lower on grazed than protected sites, but otherwise soils were similar (Marquiss and Lang 1959).
Additional studies suggest plants may be more sensitive to livestock than wildlife grazing, especially when livestock use is heavy. At big sagebrush-grassland sites near Logan, Utah, plants were six times more abundant in areas grazed only by mule deer (Odocoileus hemionus) than on sites grazed by milk cows and horses. Livestock grazing was heavy and began in early spring. Mule deer mostly avoided the livestock use areas (Smith 1949). In another study in big sagebrush-grasslands in northern Utah, abundance of arrowleaf balsamroot increased with the removal of summer cattle grazing. The number of plants found on a total of 70 plots (100 ft² [9.3 m²) was 676 in 1948 and 1,696 in 1982 on mule deer winter range avoided by livestock and was 96 in 1948 and 704 in 1982 on mule deer winter range used by cattle until 1957 (Austin and Urness 1998).
Wildlife And Livestock Use
Arrowleaf balsamroot is an important food and cover source for many wildlife and livestock species including elk (Cervus canadensis), deer (Odocoileus spp.), bighorn sheep, various small mammals, and game birds. It is one of the most important early season species for wildlife. It is highly palatable and sought after from before the time of first leaf emergence through flowering. Large mammals are thought to migrate to large arrowleaf balsamroot patches for feeding (S. Monsen, USFS retired, personal communication, March 2018).
Although a small percentage of seed may be passed by large mammals, germination of digested arrowleaf balsamroot seed is unlikely. In captive feeding trials, about 10% of seeds fed to bison (Bos bison) were recovered. Neither passed nor control seeds germinated, suggesting the digestive system did not impact seed dormancy (Gokbulak 2002).
Elk, deer, and bighorn sheep. Elk, deer, and bighorn sheep feed on arrowleaf balsamroot. Feeding may be more concentrated in the winter and spring but often occurs year-round (Schallenberger 1966; Kufeld 1973; Kufeld et al. 1973; Beck and Peek 2005a).
Arrowleaf balsamroot ranked as the seventh to tenth most important plant in the summer diets of three elk populations in west-central Montana. Diets were determined from fecal samples collected from grassland to subalpine fir forest habitats from May to September. Cumulative percentage of arrowleaf balsamroot in elk diets ranged from 53 to 70% (Devoe et al. 2019). Elk and deer competed for arrowleaf balsamroot in early spring on the Pocatello Game Preserve in the Caribou National Forest. Both sought new shoots and cropped them close to the ground for a 3- to 4-week period (Hoskins and Dalke 1955). On winter ranges in the Sapphire Mountains of western Montana, arrowleaf balsamroot was consumed by mule deer and elk, but greater quantities of the plant were recovered from mule deer than from elk fecal samples. It was considered “highly preferred” mule deer winter forage (Lavelle 1986). In the Jarbidge Mountains of Nevada, arrowleaf balsamroot made up a high of 7% of spring and 4% of summer elk diets and 4% of spring and summer mule deer diets over 3 years (Beck and Peek 2005a).
Plant use by mule deer can change by site, season, or individual preference. On critical winter range in central Washington, use of arrowleaf balsamroot increased with decreased availability of antelope bitterbrush. From two seasons of fecal analyses, use of arrowleaf balsamroot was 6 to 8% where antelope bitterbrush abundance was greatest and 19% where it was least (Burrell 1982). In the Little Belt Mountains of central Montana, arrowleaf balsamroot was an important fall, but not summer or winter mule deer food (Lovaas 1958). In the Bridger Mountains of Montana, it was one of the most important year-round forb foods with almost equal use in all seasons (Wilkins 1957). On the National Bison Range (NBR) in western Montana, it provided a high proportion of spring and fall diets (25.3-42.8%). Flower heads were eaten in the spring and dry leaves and stems in the fall (Nellis and Ross 1969). Morris and Schwartz (1957), also working on the NBR, found arrowleaf balsamroot year-round in individual mule deer stomach samples. In one stomach sample taken on 6 June 1952, arrowleaf balsamroot made up 35% of stomach contents (Morris and Schwartz 1957). In a captive feeding trial, one mule deer consumed arrowleaf balsamroot any time it was presented but another mule deer refused it, suggesting that preference can be individual (Smith 1953).
Arrowleaf balsamroot is an important spring and winter food for bighorn sheep. Bighorn sheep commonly paw away snow and soil to feed on semi-decomposed leaves and roots in the winter (Buechner 1960; Kasworm et al. 1984; Wagner and Peek 2006). Plants are often grazed again in the spring. In transitional vegetation between the sagebrush and ponderosa pine zones in southern British Columbia, arrowleaf balsamroot was highly preferred. Plants were grazed in the spring and again once they resprouted following grazing damage. Use was greatest in June when the months of May through August were compared (Wikeem and Pitt 1979). In big sagebrush-grasslands in the Frank Church-River of No Return Wilderness in Idaho, arrowleaf balsamroot provided 10% of bighorn sheep diets from June to August (Elliott and Flinders 1984). Based on summer feeding observations made in the Teton range, northwestern Wyoming, arrowleaf balsamroot was one of the most frequently used bighorn sheep forages (Courtemanch and Kauffman 2008).
Diet composition and plant preference may change from year to year (Wikeem and Pitt 1991). When bighorn sheep were kept in enclosures in transitional sagebrush-ponderosa pine communities in southern British Columbia. In fecal analysis, arrowleaf balsamroot made up 88% of diets in 1977, 20% in 1978, and only a trace in 1979, even though frequency of the plant was relatively unchanged over the same time period (Wikeem and Pitt 1991).
Small mammals. A variety of small mammals feed on arrowleaf balsamroot plants and seeds. Red-tailed chipmunk (Tamias ruficaudus) use of seeds was observed in Montana (Best 1993). Adult and juvenile Columbian ground squirrels (Urocitellus columbianus) fed extensively on arrowleaf balsamroot throughout the summer in a sagebrush-grassland in Idaho’s Frank Church-River of No Return Wilderness, where it made up 20 to 59% of their diets (Elliott and Flinders 1985).
Deer mice feed heavily on arrowleaf balsamroot seeds. In bunchgrass communities in western Montana, they removed all seed provided in experiments and largely limited seedlings to protected areas (Pearson and Callaway 2008). In captive feeding trials, arrowleaf balsamroot seed ranked as the second or third most preferred food of deer mice captured in western Nevada (Everett et al. 1978).
Birds. Arrowleaf balsamroot provides important cover and a seed and insect food source for game birds. In Hells Canyon, Idaho and Oregon, arrowleaf balsamroot seeds were recovered from the crops of gray partridges (Perdix perdix) killed during the hunting season. The seeds made up 0.6% of fall and winter diets at a time when seed was likely scarce (Churchwell et al. 2004). In south-central Wyoming, arrowleaf balsamroot is common in greater sage–grouse (Centrocercus urophasianus) habitats and researchers indicate it is a likely food source (Kirol et al. 2012).
Plants are typical in rangelands used by game birds and provide important cover and nesting habitat. In shrublands in Washington County, Idaho, summer sites used by sharp-tailed grouse (Tympanuchus phasianellus) had significantly (P < 0.05) higher cover of arrowleaf balsamroot than did random sites (Saab and Marks 1992). Plants provided important nesting cover and were especially important during drought conditions when annual cover was lacking (Marks and Marks 1987). In southeastern Idaho, arrowleaf balsamroot was more frequent on greater sage-grouse nesting sites than on non-nesting sites. Plants were also associated with broods but reasons for this relationship were not investigated (Klebenow 1969). In canyon grasslands of west-central Idaho, 2 of 23 chukar (Alectorus chukar) nests were found in arrowleaf balsamroot plants (Lindbloom et al. 2003).
Insects. In addition to the insect use already mentioned in the Pollination section, arrowleaf balsamroot is a host plant for the following Lepidopterans: Bucculatrix divisa, Tebenna balsamorrhizella, T. gemmalis, Euxoa auxiliaris, Exaeretia umbraticostella, Adaina cinerascens, and Oidaematophorus occidentalis (Robinson et al. 2023).
Other. The Tiny Canyon mountain snail (Oreohelix spp.), an endemic to dry forests in the Entiat River watershed in Washington state, is commonly found in the leaf litter and near the caudex of arrowleaf balsamroot plants. The plants may serve as a refuge during fires and when snails enter a state of torpor to avoid stress from high temperatures and aridity (Gaines et al. 2011).
Livestock. Cattle, domestic sheep, and horses feed on arrowleaf balsamroot. Usage is generally greatest by sheep, and plants are most preferred in the spring (Mueggler and Stewart 1980). Horses prefer and selectively feed on the flowers (USDA FS 1937, S. Monsen, USFS retired, personal communication, March 2018). Cattle consumed entire plants in late spring but avoided dry plants on the Starkey Experimental Range near La Grande, Oregon (Holechek et al. 1982).
Utilization by sheep is generally greater than by cattle or horses. In western Montana, arrowleaf balsamroot abundance in shrublands and grasslands generally increased with cattle or horse grazing but decreased substantially with spring or early summer sheep grazing (Mueggler and Stewart 1980). On summer range in the Wasatch Mountains of northern Utah, utilization was 76% by sheep and 20% by cattle when monitored from July through September (Cook 1954).
Arrowleaf balsamroot is highly preferred by sheep. In sagebrush grasslands at the USSES, arrowleaf balsamroot was 66% of spring sheep diets (Harniss et al. 1975). Sheep use was 47% in early summer and 39% in late summer in the same general area (Harniss and Wright 1982). On big game winter range near Logan, Utah, late-June utilization by sheep was a high of 22% with moderate-intensity grazing and 71% with high-intensity grazing (Jensen et al. 1972).
Nutritional Value
Arrowleaf balsamroot is generally more nutritious in the spring than in the summer or fall. Protein, phosphorus, and fat content in the spring as measured at the USSES is considered good for livestock (Tables 2 and 3; Blaisdell et al. 1952; Elliott and Flinders 1984).
Table 2. High and low average percentage chemical composition of herbage (all aerial plant parts) of arrowleaf balsamroot (20 plants/date) monitored for 4 years at the USDA ARS Sheep Experiment Station near Dubois, Idaho (Blaisdell et al. 1952).
Date |
Dry weight (lb) |
Crude protein (%) |
Crude fat (%) |
N-free extract (%) |
Crude fiber (%) |
Ca (%) |
P (%) |
Mid- to late April |
0.007 |
29-31 |
3.4-6.4 |
32-35 |
14.7-14.9 |
0.6-0.9 |
0.4-0.6 |
Early to mid-May |
0.07-0.3 |
17-31 |
3.9-6.1 |
31-41 |
14-17 |
1.2-2.2 |
0.2-0.6 |
Late May to early June |
0.4-0.6 |
12-19 |
2.6-4.7 |
37-45 |
15-21 |
1.4-2.2 |
0.2-0.3 |
Mid-June to late June |
0.4-0.7 |
8-14 |
2.1-3.1 |
39-48 |
18-25 |
1.7-2.4 |
0.1-0.2 |
Table 3. Average chemical composition of aerial arrowleaf balsamroot plant parts growing in big sagebrush/grasslands in the Frank Church-River of No Return Wilderness in Idaho (Elliott and Flinders 1984).
Month |
Crude fiber (%) |
Crude protein (%) |
Ca (%) |
P (%) |
June |
29 |
20 |
2.45 |
0.26 |
July |
31 |
14 |
0.21 |
0.21 |
August |
29 |
10 |
0.19 |
0.19 |
In the Jarbidge Mountains of Nevada, crude protein content averaged 17% in early summer, 8% in mid-summer, and 6% in early fall over a 2-year period. Digestible energy averaged 3,479 kcal/kg in early summer, 2,777 kcal/kg in midsummer, and 2,294 kcal/kg in early fall. Only early summer protein content and digestible energy were considered adequate for elk. Calcium and potassium levels exceeded the maximum tolerable for elk (Beck and Peek 2005b).
An experimental field study found that nectar volume decreased while sugar concentration increased and total flower production increased with experimental warming treatments. The study was conducted in 2015 and 2016 in a montane meadow in Grand Teton National Park, Wyoming. Plots were warmed passively using a plastic topped, open sided chamber designed to admit precipitation. These treatments increased soil temperatures 3.2 °F (1.75 °C) in 2015 and 2.6 °F (1.43 °C) in 2016 (based on means of soil temperature differences at 5 am in warmed and control plots). The number of arrowleaf balsamroot flowers containing nectar and the nectar volume decreased in warmed plots with the difference in nectar volume significant in 2015 (P = 0.024). Sugar concentration increased in warmed plots in both years and (significantly in 2015, P = 0.016). Warming significantly increased the total number of flowers produced (P = 0.05 in 2015) and (P = 0.1 in 2016), likely by reducing number of frost-killed buds (McCombs et al. 2022).
Ethnobotany
Arrowleaf balsamroot has antibacterial (Matsuura et al. 1996) and antifungal properties (McCutcheon et al. 1994). It was used extensively by western tribes as food and medicine and was also important ceremonially.
Foods. Western Indians ate young shoots and stems, mature seeds, and roots of arrowleaf balsamroot. At least some portion of the plant was noted as an important food source of the Atsugewi, Blackfoot, Cheyenne, Flathead, Goshute, Miwok, Nez Perce, Okanagan-Colville, Paiute, Secwepemc, Thompson, and Ute peoples (Chamberlin 1911; Stubbendieck et al. 1986; Moerman 2003). Young leaves and stems were eaten raw or cooked. Juice from stems was sucked to quench thirst, and stems were chewed to ease hunger pains. Seeds were often ground into a flour, which was often stored or mixed with animal fat, nuts, and berries and eaten as cakes or porridge. Roots were used extensively. They were roasted and eaten immediately, strung on a line and eaten later, or used in trading (Moerman 2003).
The plant was also useful for other aspects of cooking. Seeds were used as an oil for cooking (Chamberlin 1911), leaves were used in the cooking of camas (Camassia quamash) bulbs (Johnston 1970), and leaves provided a clean space to lay or dry clean fish (Moerman 2003).
Aboriginal people of British Columbia harvested and ate large quantities of roots (Mullin et al. 1997; Turner et al. 2000). Ideal plants for harvesting had 6 to 12 leaves and a taproot about the size of a carrot. Large plants with 40 to 50 leaves were considered “mother” plants and purposely not harvested to ensure future plant recruitment. Women and children were the primary root harvesters and used pointed wood or antler digging sticks. Processing and cooking the roots was also primarily the job of women (Turner et al. 2000). Roots were pit cooked or steamed for long periods in earthen ovens (Mullin et al. 1997). Interior Salish women would abstain from sex when digging or cooking roots, and men were not allowed near the cooking pits. Prayers were offered when eating the first roots of the season (Turner et al. 2000).
Traditional pit–cooking increased the nutritional value of roots. Protein content of roots harvested in May ranged from 2% for fresh roots and 4% for pit-cooked roots. Fiber content was 52% for fresh unpeeled, 25% for fresh peeled, 36% for unpeeled pit-cooked, and 43% for peeled pit-cooked roots. Pit-cooked roots were high in fructose (21-22%) and had a total energy range of 202 to 211 kcal/100 g. (Mullin et al. 1997). In experiments replicating Interior Salish pit-cooking methods, the root energy provided by simple sugars was increased 250% by cooking that converted inulin to fructose (Peacock 2008).
Medicines. Arrowleaf balsamroot was taken internally and applied externally to treat ailments and wounds (Chambers et al. 2002; Moerman 2003). A poultice from the roots was used on burns, wounds, cuts, and bruises. Roots also stimulated salivation and bronchiole activity, which eased symptoms of tuberculosis. Leaves have antibacterial properties and were commonly used to treat infections. Smoke from the leaves was used to treat aches and pains associated with illnesses. The Secwepemc still use the roots to treat skin ailments and infections (Chambers et al. 2002).
Roots and leaves were chewed or pounded into a poultice to treat and prevent infection of blisters, bruises, boils, burns, and other wounds or sores by the Blackfoot, Gosiute, Kootenai, Okanagan-Colville, Sanpoil, Shuswap, and other Flathead Indian tribes and aboriginal peoples of British Columbia (Chamberlin 1911; Turner et al. 2000; Chambers et al. 2002; Moerman 2003). Blackfoot inhaled smoke from the roots for body aches (Moerman 2003). Cheyenne used a decoction or smoke of the plant for stomach pains, labor pains, headaches, colds, and fevers, and chewed roots for sore throats, mouths, and teeth (Stubbendieck et al. 1986; Moerman 2003). Flathead Indians used a root infusion to treat whooping cough and tuberculosis (Moerman 2003). The Miwok used a root decoction to treat headaches and to induce profuse perspiration as a way to treat rheumatism. Okanagan-Colville put leaves on glowing coals to induce profuse sweating. Paiute tribes used the root in various ways to treat stomach aches, insect bites, swellings, syphilitic sores, and tuberculosis. Shoshoni used the root as an eyewash and in the treatment of syphilitic sores. The Paiute and Washoe burned the root to fumigate sick rooms. Thompson ate the seeds to treat dysentery and large quantities of young shoots for a sleep aid (Moerman 2003).
Ceremonies and other. Before introduction of the horse, the Blackfoot burned arrowleaf balsamroot as an incense to prepare the runner who would herd buffalo toward a jump. The runner would bathe in the smoke from dry roots. This ceremony was thought to help the runner make the long distances of more than 20 miles a day. Arrowleaf balsamroot was also used in the transfer ceremony of the Beaver bundle (Moerman 2003).
In the winter, the Okanagan stuffed large arrowleaf balsamroot leaves into their moccasins for warmth (Chambers et al. 2002). Leaves were also used to teach children how to walk softly. Young Okanagan boys would wrap leaves around their feet and challenge themselves to make long distances without tearing the leaves, which was an exercise in being stealthy in the woods. Fibrous dried roots were gathered into tight bundles and used as a slow burning match for building fires (Chambers et al. 2002).
Horticulture
A native plant gardening guide for the Pacific Northwest indicates that arrowleaf balsamroot is easy to grow from seed on the east side of the Cascade Range but does less well on the west side. Plants grow well in rocky sites with wet winters and dry summers. In dry gravelly soils with good drainage, arrowleaf balsamroot may also be grown on the west side of the Cascades (Kruckeberg et al. 2019).
Revegetation Use
Arrowleaf balsamroot is an important revegetation species for many reasons: it provides forage for wildlife, livestock, and pollinators; it is long-lived and mature plants are competitive with nonnative species; and it is generally persistent and tolerant of periodic above-ground disturbances (Horton 1989; Stevens et al. 1996; Stevens and Monsen 2004). For these reasons it is a choice for restoration, and it has been used successfully in revegetation of upper elevation big sagebrush, quaking aspen, oak brush, and pinyon-juniper sites (S. Monsen, USFS retired, personal communication, March 2018).
As a long-lived, mid- to late-seral species, initial plant growth is slow and even with the best growing conditions it can take 3 or more years to reach reproductive maturity and develop considerable cover (Stevens and Monsen 2004). Therefore the use of arrowleaf balsamroot in restoration must be carefully considered based on long-term restoration planning, goals, and strategies. It is not a species that will provide immediate soil stabilization (Shaw and Monsen 1983).
A combination of factors, which include predation by insects and rodents and seeding on sites where arrowleaf balsamroot is not adapted, may explain sporadic stand establishment (Stevens and Monsen 2004). Seeding has often failed at sites outside of the species’ range. The most successful seedings have occurred in upper-elevation sagebrush and mountain brush communities (Stevens and Monsen 2004; S. Monsen, USFS retired, personal communication, March 2018).
Developing A Seed Supply
For restoration to be successful, the right seed needs to be planted in the right place at the right time. Coordinated planning and cooperation is required among partners to first select appropriate species and seed sources and then properly collect, grow, certify, clean, store, and distribute seed for restoration (PCA 2015).
Developing a seed supply begins with seed collection from native stands. Collection sites are determined by current or projected revegetation requirements and goals. Production of nursery stock requires less seed than large-scale seeding operations, which may require establishment of agricultural seed production fields. Regardless of the size and complexity of any revegetation effort, seed certification is essential for tracking seed origin from collection through use (UCIA 2015).
Seed Sourcing
Arrowleaf balsamroot plants occur on a variety of soils and in many precipitation zones. Population differences related to palatability, seed production, and drought tolerance exist, as do locally adapted ecotypes and hybrids (Stevens and Monsen 2004). It is therefore recommended that planting sites and seed collection sites be matched (Wasser 1982; Stevens and Monsen 2004).
Because empirical seed zones are not currently available for arrowleaf balsamroot, generalized provisional seed zones developed by Bower et al. (2014), may be used to select and deploy seed sources. These provisional seed zones identify areas of climatic similarity with comparable winter minimum temperature and aridity (annual heat:moisture index). In Figure 7, Omernik Level III Ecoregions (Omernik 1987) overlay the provisional seeds zones to identify climatically similar but ecologically different areas. For site-specific disturbance regimes and restoration objectives, seed collection locations within a seed zone and ecoregion may be further limited by elevation, soil type, or other factors.
The Western Wildland Environmental Threat Assessment Center’s (USFS WWETAC 2024) Threat and Resource Mapping (TRM) Seed Zone application provides links to interactive mapping features useful for seed collection and deployment planning. The Climate Smart Restoration Tool (Richardson et al. 2020) can also guide restoration planning, seed collection, and seed deployment, particularly when addressing climate change considerations.
Occurrence Map
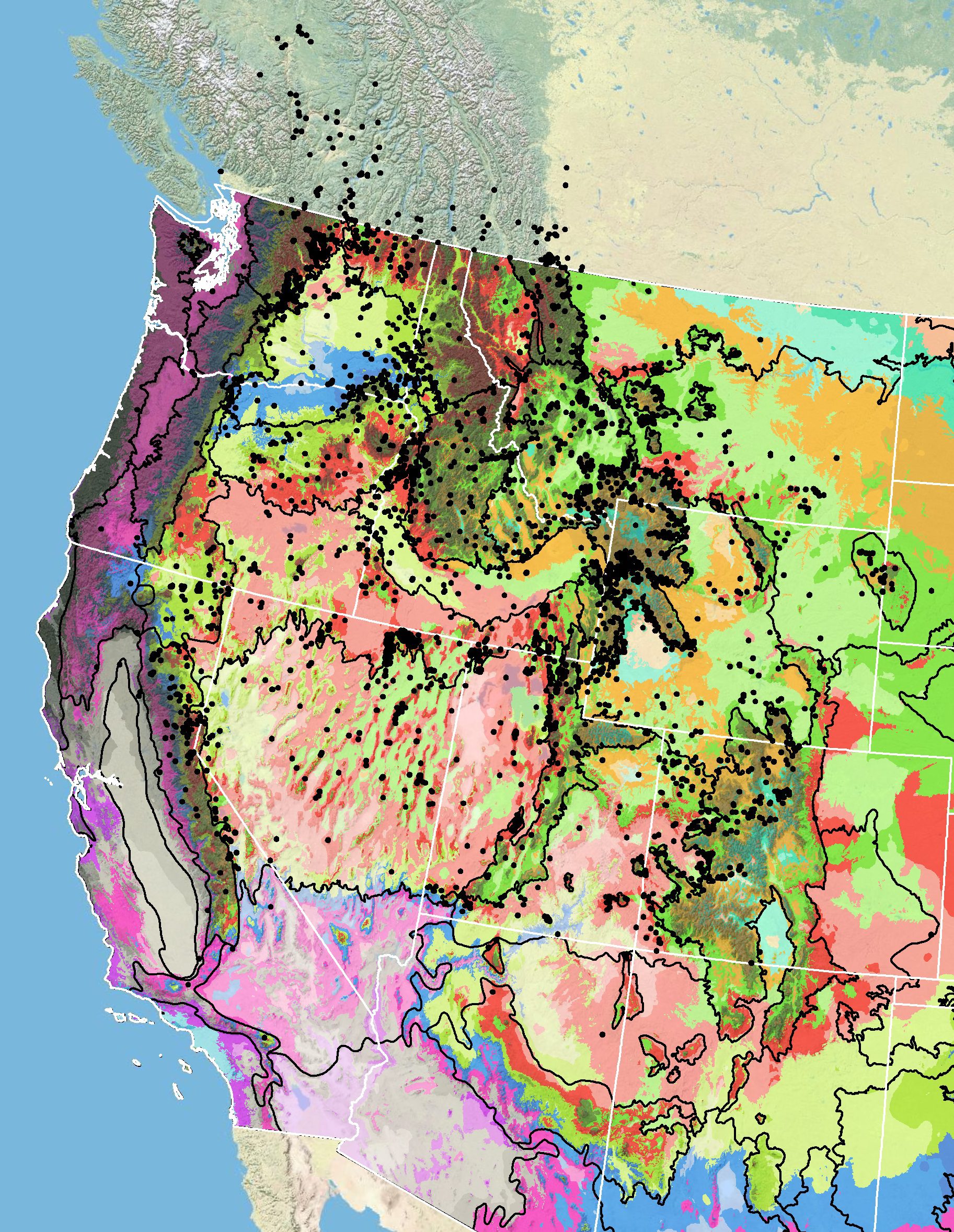
Figure 7. Distribution of arrowleaf balsamroot (black circles) based on geo-referenced herbarium specimens and observational data from 1870-2016 (CPNWH 2017; SEINet 2017; USGS 2017). Generalized provisional seed zones (colored regions) (Bower et al. 2014) are overlain by Omernik Level III Ecoregions (black outlines) (Omernik 1987; USDI EPA 2018). Interactive maps, legends, and a mobile app are available (USFS WWETAC 2024). Map prepared by M. Fisk, USGS.
Releases
As of early 2018, there were no arrowleaf balsamroot germplasm releases.
Wildland Seed Collection
The majority of arrowleaf balsamroot seed used for revegetation is wildland collected (S. Monsen, USFS retired, personal communication, March 2018). Seed is typically harvested between mid-May and late July (Jorgensen and Stevens 2004a), but exact harvest dates will vary with weather and site conditions (Pechanec et al. 1937; Amsberry and Maron 2006; USDI BLM SOS 2017). Seeds ripen relatively uniformly and are harvestable when the inflorescence is dry and seeds are dark brown or black (Fig. 8; Skinner 2004). Harvest plans should include methods to avoid livestock use areas, periodic phenology checks to estimate seed predation, and inspections of flower heads for extensive insect or frost damage (Wasser 1982; Skinner 2004).
Often a high percentage of seed fails to ripen or fill, making periodic checks of seed quality a critical part of efficient wildland seed monitoring. Seed failures can be the result of insect predation, weather, or a combination of factors. Failures vary from stand to stand and year to year. Regardless of the causes of poor seed development, careful inspection of flower heads and seeds allows collectors to concentrate their efforts on high-quality stands. As flowers are maturing, collectors can cut across the flower head horizontally to see how many flowers are producing viable seeds, which will appear white and plump. This will allow collectors to return only to those sites with the greatest percentages of filled seed. If sites are visited as seed is maturing, seed fill can be evaluated by cutting the seeds, because filled and non-filled seeds appear nearly identical externally. Empty seeds will be papery on the inside and often collapse under the pressure of the knife. Stands where plants are producing flower heads with about 50% of seed filled are considered acceptable for collection (S. Monsen USFS retired, personal communication, March 2018).
Wildland Seed Certification
Verification of species and tracking of geographic source is necessary whether wildland seed is collected for immediate project use or as stock seed for cultivated increase. This official Source Identification process can be accomplished by following procedures established by the Association of Official Seed Certifying Agencies (AOSCA) Pre-Variety Germplasm Program (Young et al. 2020; UCIA 2015). Wildland seed collectors should become acquainted with state certification agency procedures, regulations, and deadlines in the states where they collect.
If wildland-collected seed is to be sold for direct use in ecological restoration projects, collectors must apply for Source-Identified certification prior to making collections. Pre-collection applications, site inspections, and species and seed amount verification are handled by the AOSCA member state agency where seed collections will be made (see listings at AOSCA.org).
If wildland seed collected by a grower or private collector is to be used as stock seed for planting cultivated seed fields or for nursery propagation (See Agricultural Seed Field Certification section), detailed information regarding collection site and collecting procedures must be provided when applying for certification. Photos and herbarium specimens may be required. Germplasm accessions acquired within established protocols of recognized public agencies, however, are normally eligible to enter the certification process as stock seed without routine certification agency site inspections. For contract grow-outs, however, this collection site information must be provided to the grower to enable certification.
Figure 8. Appearance of arrowleaf balsamroot plants near seed harvest time. Photo: USDI BLM WY050 SOS.
Collection Timing
Seed is harvested when flowers are dry and the achenes are dark brown or black and not dented by fingernail pressure (Skinner 2004; Camp and Sanderson 2007). While seed size is a good quick visual indicator of viability (Cane 2005), a check of fill provides a better estimate of fresh seed viability. Proper timing of harvests is critical to avoid seed loss to birds and small mammals (Skinner 2004). Insect predation of flower heads is common, and immature seed is retained longer in the flower heads than mature seed (Stevens and Monsen 2004). Flower heads are also eaten by wildlife and livestock, so areas receiving heavy spring use may not be good harvest sites (Wasser 1982).
From 17 years of collections made by the BLM Seeds of Success (SOS) field personnel, the most common harvest dates were in June or July (USDI BLM SOS 2017, 2024). The SOS teams made 122 collections in 10 western states from 2002 to 2023, 56 of these were made in June and 45 were made in July. Collection crews reported an earliest harvest date of 10 May 2015 at an elevation of 4,729 ft (1,441 m) in Crook County, Oregon. The latest harvest date reported was 31 October 2012 from an elevation of 6,369 ft (1,941 m) in Churchill County, Nevada. Earlier harvest dates were generally associated with lower elevations, and later harvest dates were associated with higher elevations. In the single year (2021) with the most collections made (18), the earliest collection date was June 7 made at 4,361 ft (1,329 m) in Malheur County, Oregon, and the latest harvest date was July 15 made at 7,300 ft (2,225 m) in Mono County, California. (USDI BLM SOS 2017, 2024). In western Montana, seed ripening was 3 weeks earlier at 3,610 ft (1,100 m) than at 6,000 ft (1,825 m) elevation (Amsberry and Maron 2006). During drought conditions near Dubois, Idaho, plants began growing about 1 month earlier than normal (March 25). Plants were dry by May 1 and produced some flowers but little seed. In this year, annual precipitation was 2.5 in (63 mm) less and winter precipitation was 1.5 in (38 mm) less than average (Pechanec et al. 1937). Seed that was wild collected from southern interior British Columbia was also collected earlier from more southern and lower elevation sites than from more northern and higher elevation sites. The elevation range for seed collection was 1,400 to 3,000 ft (425-900 m), and difference in the earliest and latest collection dates was about 10 days (Monthony et al. 2020).
Collection Methods
Seed ripens relatively uniformly and is easily collected by hand stripping, beating flower heads into a container, cutting stems, or plucking intact flower heads (Fig. 9 and 10; Stevens and Monsen 2004; Camp and Sanderson 2007). When nearly pure stands of arrowleaf balsamroot occur on level terrain, combines or other reel-harvesters may be used for wildland harvests (Shaw and Monsen 1983). Seed vendors have developed modified reel-harvesters for use on all-terrain vehicles (S. Monsen, USFS retired, personal communication, March 2018). Van Epps and Stevens (2004) suggested that removing or flagging rocks and other vegetation in a wildland setting may allow for use of head stripper or beater equipment to efficiently harvest large amounts of wildland seed. Recommendations are for wildland seed collections to remove less than 5% of the seed within a population (Bowen et al. 2006).
Figure 9. Collection of arrowleaf balsamroot seed heads. Photo: USDI BLM WY030 SOS.
Several collection guidelines and methods should be followed to maximize the genetic diversity of wildland collections: 1) collect seed from a minimum of 50 randomly selected plants; 2) collect from widely separated individuals throughout a population without favoring the most robust or avoiding small stature plants; and 3) collect from all microsites including habitat edges (Basey et al. 2015). General collecting recommendations and guidelines are provided in online manuals (e.g., ENSCONET 2009; USDI BLM SOS 2023).
It is critical that wildland seed collection does not impact the sustainability of native plant populations. Collectors should take no more than 20% of the viable seed available at the time of harvest (USDI BLM SOS 2023). Additionally, care must be taken to avoid the inadvertent collection of weedy species, particularly those that produce seeds similar in shape and size to those of arrowleaf balsamroot.
Collection Rates
Monsen (USFS retired, personal communication, March 2018) estimated 150 lbs (68 kg) of bulk seed could be hand collected by 2 or 3 people over 2 or 3 days.
Figure 10. Collection of arrowleaf balsamroot seeds. Note the appearance of petals at collection time. Photo: USDI BLM OR130 SOS.
Post-Collection Management
Seeds and seed head materials may be somewhat green at the time of collection, so should be dried at room temperature for 2 to 4 weeks before cleaning (Camp and Sanderson 2007). Soon after harvesting, thorough drying of the seed by allowing for air movement through the seeds and flower heads is important to maintaining seed viability (S. Monsen, USFS retired, personal communication, March 2018). Seeds should be kept in a location free of rodents. To limit seed loss to insects collected with the seed head, dry seed can be treated with an insecticide, put into a freezer, or both (S. Monsen, USFS retired, personal communication, March 2018; Brown and Allen 2023).
Seed Cleaning
Because arrowleaf balsamroot seed is large, smooth, and without appendages, the cleaning process is simple. If there are lightweight or empty seeds in the collections, they are easily separated from filled seeds using gravity, wind, or flotation cleaning techniques (Stevens and Monsen 2004). The basic steps for seed lot cleaning are running dry seed through a Barley debearder and then an air- or fan-screen separator (Stevens et al. 1996; Jorgensen and Stevens 2004a).
There are several multi-step cleaning procedures for small seed lot collections of intact flower heads. Hand cleaning can be done by crushing flower heads and rubbing them over a hand screen to separate the flowers and seeds. An air column separator can be used to eliminate trash and damaged seeds (Skinner 2004).
The Bend Seed Extractory developed two cleaning procedures for small (1- 2 lbs [0.45-0.9 kg]) seed lots:
1. Separate the seeds from flower heads in a hand tumbler. Next, air-screen seeds using an office clipper with a size 12, round, top screen and 1/22, round, bottom screen, set at medium speed with air. Use an air column separator for final seed cleaning and separation (Barner 2009a).
2. Separate seeds from the flower heads using a Westrup Model LA-H laboratory brush machine with a #40 mantel and a speed of 3. Finish seeds by air screening with an office Clipper using a size 13, round top screen and size 5 ½, round bottom screen, set at medium speed with air (Barner 2009b).
Seed Storage
Arrowleaf balsamroot seed is orthodox (SER, INSR, RBGK 2023). Reported storage conditions for dry, clean seed were temperatures of 33 to 40 °F (0.6–4 °C) and humidity of 40% (Skinner 2004; Barner 2009b).
Seed can be stored for 5 to 7 years without significant loss of viability, but seeds are susceptible to insect damage so treatment with a pesticide prior to storage is necessary (Stevens et al. 1996; Jorgensen and Stevens 2004a). When hand-cleaned, dry seed (≥ 85% purity) was stored in an open warehouse in a semi-arid location where the low temperature reached -21 ºF (-29 ºC) and high temperature reached 101 ºF (38 ºC), germination was 42% after 3 years, 35% after 5 years, 20% after 7 years, and 1% after 10 years of storage (Stevens et al. 1981).
Seed Testing
There are Association of Official Seed Analysts (AOSA) guidelines and testing procedures available for testing arrowleaf balsamroot seed.
Viability Testing
Association of Official Seed Analysts (AOSA) guidelines for TZ testing procedures and staining evaluations are available (AOSA 2010). Seed is imbibed on moist media overnight at 68 to 77 °F (20-25 °C). Seed is then cut longitudinally, leaving the distal seed end intact. Seed is exposed to a 0.1% TZ concentration for 6 hours or overnight at 86 to 95 °F (30-35 °C). Staining is observed by removing the embryo from the achene or spreading the seed halves apart. Slight damage to the radicle tip and cotyledons less than half stained on areas away from the cotyledon point of attachment are acceptable. Viable embryos are evenly stained (AOSA 2010).
Purity Testing
Minimum and maximum seed weights for laboratory purity testing are 25 g and 0.5 lb (250 g), and approximately 100 seeds weigh 1 gram (AOSA 2023).
Germination Testing
Germination testing rules were informed by several germination studies, many of which reported low germination at temperatures above 36 °F (2 °C) without prechilling for more than 70 days (Evans et al. 1982; Mogensen et al. 2001; Jorgensen and Stevens 2004b; Sheley and Half 2006). In-depth investigations of seed germination made by Kitchen and Monsen (Kitchen and Monsen 1996) led Kitchen et al. (1998) to develop the first testing rules.
Association of Official Seed Analysts (AOSA) germination testing rules now exist for arrowleaf balsamroot seed. The rules require prechilling at 36 °F (2 °C) for 70 days before testing germination at 41 to 59 °F (5-15 °C). First emergence counts are made at 7 days and last counts at 14 days. A tetrazolium (TZ) test can be used to determine the viability of ungerminated seed following the standard germination test (AOSA 2023).
Germination Biology
There are many reports of low germination of arrowleaf balsamroot seed at temperatures above 36 °F (2 °C) without cold stratification of 70 days or more (Evans et al. 1982; Mogensen et al. 2001; Jorgensen and Stevens 2004b; Sheley and Half 2006), but afterrippening of seed collections and site conditions (elevation) of the parent population can impact the duration of cold stratification needed to get seed to germinate.
Kitchen and Monsen (1996) conducted laboratory and field tests on seed collected in May and June from 7 populations in Utah and Idaho (Table 4). Moist prechilling (34-36 °F [1-2 °C]) was required to break dormancy and induce normal seedling growth for all collections. Germination increased with increased duration of wet prechilling (0-12 wks) but varied among collections. Afterripened seed (stored 3 yrs at 68 °F [20 °C]) germinated better than recently harvested seed following 4- and 8-week prechilling treatments (Kitchen and Monsen 1996).
Brown and Allen (2023) conducted a study of how elevation influences the dormancy and germination of arrowleaf balsamroot seed, which was collected from high (8,000 ft [2,400 m), medium (6,600 ft [2,000 m]), and low (5,200 ft [1,600 m]) elevations. Seed was cold stratified in a dark incubator at 36 °F (2 °C) for 4, 8, 12, 16 or 20 weeks after which is was transferred to an incubator set to alternating temperatures of 50 °F (10 °C) and 68 °F (20 °C) associated with a 12-hour photoperiod. All seed required at least 16 weeks of cold stratification to reach 50% germination or more, and most germination occurred during the cold treatments. No seed collections reached 50% germination in the 4, 8, or 12 week cold stratification treatments. Low- and mid-elevation seeds reached higher germination percentages during cold stratification than did high-elevation seed. Although all elevations had high percentages of germination (>80%) at 16 and 20 weeks of cold stratification, germination of low-elevation seed was significantly higher than high-elevation seed (P < 0.001), and germination in post-chill incubation was faster for low- and mid-elevation seed than high-elevation seed (Brown and Allen 2023).
Wildland Seed Yield And Quality
Post-cleaning seed yield and quality of seed lots collected in the Intermountain region are provided in Table 5 (USFS BSE 2017, 2023). The data indicates that arrowleaf balsamroot seed can generally be cleaned to high levels of purity. Seed fill of fresh seed is variable but viability of filled seed is generally high.
Arrowleaf balsamroot seeds are large, averaging 49,608 seeds/lb (110,240/kg) (Table 5; USFS BSE 2017, 2023). Other sources report 53,664 to 81,949 seeds/lb (119,253-182,109 seeds/kg), a range similar to that reported in Table 5 (Stevens et al. 1996; Jorgensen and Stevens 2004a; Skinner 2004; USFS GBNPP 2014; SER, INSR, RBGK 2023).
Table 5. Seed yield and quality of arrowleaf balsamroot seed lots collected in the Intermountain region, cleaned and evaluated by the Bend Seed Extractory. Viability was tested by the Oregon State Seed Laboratory or the USFS National Seed Laboratory (USFS BSE 2017, 2023).
Characteristic |
Mean |
Range |
Samples (#) |
Bulk weight (lbs) |
3.04 |
0.25-26.66 |
63 |
Clean weight (lbs) |
0.67 |
0.005-9.66 |
63 |
Clean–out ratio |
0.22 |
0.01-0.79 |
63 |
Purity (%) |
98 |
89-99 |
63 |
Fill (%)¹ |
85 |
45-97 |
63 |
Viability (%)² |
94 |
79-98 |
50 |
Seeds/lb |
49,608 |
23,000-80,710 |
63 |
Pure live seeds/lb |
44,696 |
21,404-63,160 |
50 |
¹ 100 seed X–ray test
²Tetrazolium chloride test
Marketing Standards
Acceptable seed purity, viability, and germination specifications vary with revegetation plans. High purity and viability are required for precision seeding equipment used in nurseries and agricultural seed fields. Lower purity may be acceptable for some wildland seeding techniques.
Seed is physiologically dormant to prevent fall and summer germination (Kitchen 1994), and maximum germination potential may not be realized for 2 to 3 months after harvesting (Stevens et al. 1996). Marketing standards recommended by ((Stevens et al. 1996; Jorgensen and Stevens 2004a; Stevens and Monsen 2004) are 90 to 99% purity, 40% or greater germination, and 80 to 90% viability (Jorgensen and Stevens 2004a; Stevens and Monsen 2004).
Agricultural Seed Production
Although cultural practices for producing seed have been investigated, and seed has been produced commercially (Shock et al. 2012; Tilley et al. 2012; BFI Native Seeds 2017), arrowleaf balsamroot seed production fields are rare. This is at least partly due to slow plant maturation and adequate wildland seed harvests (S. Monsen, USFS retired, personal communication, March 2018).
Once established, stands can be harvested for 20 to 30 years, provided seed fields remain certified (Young et al. 2003). Germination and establishment can be slow and erratic, and plants take at least 3 years to produce seed (Stevens et al. 1996; Ogle et al. 2011; Tilley et al. 2012). Seed production yields of 75 to 125 lbs/ac (187-313 kg/ha) at 90% purity are typical (Stevens et al. 1996). Arrowleaf balsamroot seed production fields were established successfully in a dryland farming region in Nephi, Utah. These stands produced flowers by the third year, and although pollinators were thought to be scarce in this grassland–dominated area, seed production and yield were good (S. Monsen, USFS retired, personal communication, March 2018).
Agricultural Seed Certification
In order to minimize genetic changes in specific accessions of native species when increased in cultivated fields, it is essential to track the geographic source and prevent inadvertent hybridization or selection pressure. This is accomplished by following third party seed certification protocols for Pre-Variety Germplasm (PVG) as established by the Association of Official Seed Certification Agencies (AOSCA). AOSCA members in the U.S., Canada, and other countries administer PVG requirements and standards that track the source and generation of planting stock. Field and cleaning facility inspections are conducted to monitor stand establishment, proper isolation distances, control of prohibited weeds, seed harvesting, cleaning, sampling, testing, and labeling for commercial sales (UCIA 2015; Young et al. 2020).
Seed growers apply for certification of their production fields prior to planting and plant only certified stock seed of an allowed generation (usually less than four). The systematic and sequential tracking through the certification process requires preplanning, knowing state regulations and deadlines, and is most smoothly navigated by working closely with state certification agency personnel. See the Wildland Seed Certification section for more information on stock seed sourcing.
Site Preparation
Weed-free beds are best for establishment of seed production stands (Tilley et al. 2012). Protection of seeds from predation is necessary. In establishment of fall-seeded production fields in 2010 at Oregon State University’s Malheur Experiment Station near Ontario, Oregon, emergence of arrowleaf balsamroot was best (66.7%) for untreated seed covered with a thin layer of sawdust and further protected by row cover (Shock et al. 2012). Emergence in the same year was poorest (25.5%) when seed was covered with sawdust but not protected by row cover. For seeding done in the following year (2011), results were similar but emergence was best (69.9%) for seed pretreated with a fungicide mixture, covered with sand and sawdust, and protected by row cover. Emergence was poorest (37.9%) for seed not protected by row cover (Shock et al. 2012).
Seed Pretreatments
Afterripening, laboratory stratification, and chemical pretreatments can improve arrowleaf balsamroot germination, but are less effective than fall seeding to provide natural stratification. An afterripening period of 1 month to several years combined with cool moist stratification improves germination (Jorgensen and Stevens 2004a), but emerging radicles of artificially stratified seeds are easily damaged (Young and Evans 1979). Ethylenne exposure was shown to increase seed germination from 2.5 to 25% (Bowen et al. 2006: Chambers et al. 2006), but not as much as natural stratification after fall planting nontreated seeds in eastern Oregon (Shock et al. 2012). At field locations, where 3 months of continuous snow cover is assured after fall seeding, dormancy is overcome naturally (Wasser 1982).
Weed Management
Established plants are quite competitive, but if weeds become problematic they can be controlled by hand pulling, cultivation between rows, or with pre-emergent or targeted post-emergent herbicide treatments (Stevens et al. 1996; Tilley et al. 2012). Hand weeding is recommended until arrowleaf balsamroot plants can be identified, generally after the first year’s growth (Stevens et al. 1996).
Seeding
Fall seeding into hills that are 12 in (30 cm) apart with 4 to 5 seeds/hill or in rows that are 36 to 42 in (91-107 cm) apart with 20 PLS/linear ft (65 PLS/linear m) is recommended for seed production stands (Stevens et al. 1996; Tilley et al. 2012). The recommended distance between rows will allow for between row cultivation (Tilley et al. 2012). Seeds can be planted by hand or using a single-row seeder (Stevens et al. 1996). Drill seeding or using some other method to cover seeds is important (Horton 1989; Tilley et al. 2012). Seeds generally should be planted at 0.5- to 1-in (1.3-2.5 cm) depths, but ideal seeding depth may vary with site conditions (Wasser 1982; Tilley et al. 2012). Wasser (1982) recommended a burial depth of 0.5 in (1.3 cm) on medium-textured soils or moist sites and 1 in (2.5 cm) on coarse-textured soils or dry sites (Wasser 1982).
In experiments conducted in Utah, researchers were successful in establishing a dense stand of arrowleaf balsamroot designed to produce transplant stock for seed production fields (Jones and Whittaker 2011). Using nursery bedding equipment, they created a flat planting surface and seeded at 6-in (15 cm) row spacings. A dense stands was established in spring 2010 after fall seeding in 2009. Plants were to be lifted after 3 to 4 years of growth and then transplanted into a production field setting (Jones and Whittaker 2011). Reports of the later transplanting phase of the project were not available.
Establishment And Growth
Seedling emergence may continue for up to 3 years after seeding (Stevens et al. 1996; Stevens and Monsen 2004). After emergence, young plant growth is slow. It can take 3 to 6 years for plants to reach reproductive maturity, but plants can produce harvestable seed crops for 20 to 30 years (Stevens 2004b; Ogle et al. 2011; Tilley et al. 2012). Fertilizers are not recommended for establishment or growth of seed production fields (Tilley et al. 2012).
Irrigation
Seed production fields need 15 to 20 in (380-510 mm) of water each year, so depending on the field location and annual weather variations, irrigation may be necessary (Stevens et al. 1996). Irrigation can be important during the seedling establishment phase or when spring soils are dry but should be avoided (Stevens et al. 1996) or delivered in a way other than by overhead methods during the pollination period (Tilley et al. 2012). Over saturation or prolonged saturation should be avoided (Stevens et al. 1996; Tilley et al. 2012).
Pollinator Management
Bees are considered essential to agricultural production of arrowleaf balsamroot seed (see Pollination section). Cane and Love (2016) found that 15 of 38 managed bee genera pollinated arrowleaf balsamroot in wildland settings. Easily managed mason bees (Osmia california and O. montana) readily pollinate flowers and are available by the thousands from businesses that trap nesting bees from the wild. Bees can usually be provided to seed growers within a year of requesting them (Cane 2012). Research suggests at least 165 mason bees (O. californica) are needed to pollinate a 2.5-ac (1 ha) production field of 12,000 plants/ac (30,000/ha). Nesting shelters for the bees should be widely spaced throughout seed production fields (Cane 2005).
Pest Management
Arrowleaf balsamroot is host to a number of fungi, rust, and insect pests. Heavy seed losses from pathogens or diseases have been reported, and seed damage from insects and rodents is common. Insecticides are recommended for seed production fields, but proper timing to avoid harming pollinators is crucial (Stevens and Monsen 2004).
The following fungi will utilize arrowleaf balsamroot as a host: Cylindrosporium balsamorrhizae, Erysiphe cichoracearum, Pleospora balsamorhizae, P. chlamydospora, P. herbarum, P. njegusensis, Puccinia balsamorrhizae, P. acroptili, and Typhula spp. (Bruckart et al. 2012; Farr and Rossman 2023).
The following insects have been collected from arrowleaf balsamroot plants: adult black grass bugs (Irbisia brachycera, I. cascadia, I. fuscipubescens, I. serrata, and I. shulli) (Schwartz 1984) and Ilnacorella argentata (Schwartz and Scudder 2000). Lace bugs (Corythucha spp. [Tingidae, Heteroptera]) feed on plants (Gibson 1918). Anguillulina balsamophila forms leaf galls and stunts the growth of heavily infested plants (Goodey 1948). Nymph and adult forms of grasshoppers (Buckellacris chilcotinae) feed on plants (Rehn and Rehn 1945), which are also a favorite of Mormon crickets (Anabrus simplex) (Vallentine 1989).
Seed production can be severely impacted by rodent harvests (Stevens and Monsen 2004). Deer mice preyed heavily on seeds in experiments conducted in bunchgrass communities of western Montana. In the summer and fall of one year, all provided seed was removed by deer mice and seedlings were limited to areas protected from deer mice (Stevens and Monsen 2004; Pearson and Callaway 2008). For more on potential predation effects, see Grazing Response in Disturbance Ecology and Wildlife and Livestock Use sections.
Seed Harvesting
Good seed production is generally reached once stands are 3 to 6 years old (Stevens et al. 1996). Seeds are usually ripe in late May or early June when flower petals have dried. Close monitoring of seed maturation is important because filled seeds are dispersed first (Stevens et al. 1996). Insect-damaged and immature seeds, which may appear normal and viable, are retained in the flower heads and can be harvested mistakenly (Tilley et al. 2012).
Stands will produce harvestable seed crops for up to 30 years. However, some stand maintenance may be necessary to retain seed certification status for the lifespan of the stand (Young et al. 2003). Seed can be harvested by hand beating the stems into a container (Stevens et al. 1996), or using vacuum-type or reel-type harvesters (Stevens et al. 1996; Jorgensen and Stevens 2004a; Tilley et al. 2012).
Nursery Practice
Because arrowleaf balsamroot is a slow-growing species, production and use of transplant materials for seed production and in wildland settings is appealing. Reports suggest that successful establishment from transplanting bareroot or wilding stock is low (Stevens 2004a), and careful plant handling is required because of the taproot (Tilley et al. 2012). See the Seed Pretreatments section for strategies to encourage seed germination. Growth in outdoor nurseries can prevent over watering, which plants do not tolerate. Mold is a reported problem for arrowleaf balsamroot in growth chambers (Bowen et al. 2006).
Container stock has been grown successfully outdoors in Pullman, Washington (Skinner 2004). To grow plugs, wildland seed collected near the outdoor nursery site was planted into soil-filled containers. Seeds were lightly covered with soil and a thin layer of pea gravel, watered deeply, and placed outdoors in late November or early December. Additional watering was done only during extended warm, dry periods. Seedling emergence began in March and continued for about 3 weeks. Aboveground seedling growth was slow, but taproots grew more quickly. The active growing phase was 1 to 2 months, and total growing time for the production of filled containers was 5 months. It may be best to transplant the plugs before root growth fills the container. Additional hardening was not necessary for the outdoor-grown stock (Skinner 2004).
Bujak and Dougher (2020) found cone-shaped containers (164 ml [5.6 oz] Ray Leach Cone-tainer, Tangent, OR) were better than standard 4-in square pots for production based on root morphology, root dry weight, and leaf width of nursery grown arrowleaf balsamroot. Fertilization rates of 200 and 400 mg/l of nitrogen produced highest shoot dry weight, leaf width, and leaf length, while root-to-shoot ratios decreased with rates of more than 100 mg/l of nitrogen. The study did not discuss seed pretreatment or germination conditions used in the study. Study method descriptions began with germinants having radicles of at least 0.4 in (1 cm) long. These were transplanted into 150-cell plug trays filled with Sunshine mix #1 (SunGro, Agawam, MA) and grown to the cotyledon stage. After 28 days, seedlings with true primary leaves were transplanted into cone-tainers or square pots also filled with Sunshine mix #1. Plants were fertilized once per week with a water soluble fertilizer (20N:10P:20K with micronutrients) (Peat-Lite Special, Jack’s Professional, Allentown, PA) at rates of 0, 50, 100, 200, or 400 mg/l of nitrogen. Seedling growth response with increased fertilization rates was closely linked to container type, suggesting interacting effects (Bujak and Dougher 2020).
Researchers found that growth regulators can be used to encourage germination of arrowleaf balsamroot seed (Monthony et al. 2020). Gibberellic acid (5 µM GA3) induced germination of stratified seed (9 wks at 37 °F [3 °C] and 3 mos to 2 yrs at -2.2 °F [-19 °C]), and by week 12 about 42% of seed had germinated. Treatments with higher concentrations GA3 (10 µM) resulted in less germination. Karrikins (KAR2) significantly increased germination to 47% at rates of 5 µM and 60% at rates of 10 µM KAR2 compared to 14% germination of controls. KAR 11 did not work as well as KAR2. Exposing seed to both GA3 and karrikins was less effective in stimulating germination than exposure to either KAR2 or KAR11 alone. Arrowleaf balsamroot seedlings cultured on thidiazuron (10 µM TDZ)-supplemented media produced 3 to 12 new shoots from the crown suggesting another potential avenue for encouraging plantlet production (Monthony et al. 2020).
Wildland Seeding And Planting
Arrowleaf balsamroot has been rated as excellent for persistence, forage yield, and early spring palatability; good for ease of seed handling, ease of seeding, final establishment, grazing tolerance, compatibility with other species, and seed production; and poor for initial establishment, seedling growth rate, and summer palatability (Stevens and Monsen 2004).
With good seedbed preparation, proper seeding, and rodent deterrence, arrowleaf balsamroot establishes well, especially at upper elevation rangeland sites. It is well adapted for revegetation of mountain brush communities on north, east, and southwest slopes, gambel oak communities with open canopies, and mountain big sagebrush communities receiving 14 to 18 in (350-460 mm) of annual precipitation (Ogle et al. 2019).
Methods. Arrowleaf balsamroot is often recommended for inclusion in seeding mixtures to be used on cleared sites or in natural or created openings in vegetation where it is adapted. Because of its slow growth rate, it should be seeded in rows separate from rapidly developing species to reduce competition and improve growth and survival of seedlings (Shaw and Monsen 1983; Tilley et al. 2012). When seeded with rapidly establishing and growing species, it may take 10 years or more for arrowleaf balsamroot to reach reproductive maturity (Stevens and Monsen 2004). Although reproduction is delayed, established plants become large and provide good soil cover as they reach reproductive age (S. Monsen, USFS retired, personal communication, March 2018). It may be seeded with other slow-growing forbs or shrubs. Seeding arrowleaf balsamroot in alternate rows with bluebunch wheatgrass has been particularly successful (S. Monsen, USFS retired, personal communication, March 2018). Recommended seed mix rates are between 5% and 25% of the full stand rate of 20 to 25 PLS/ft² (215-269 PLS/m² ) with a 12-in (30 cm) drill row width (Wasser 1982; Kitchen 1994; USDA NRCS 2000).
Steven Monsen (USFS [retired], personal communication, March 2018) recommends drill seeding because broadcast seeding followed by chaining has a low probability of success. Others, however, found survival in post-seeding year 6 was not different on drill-seeded sites or sites where broadcast seeding was followed by a Lawson aerator, Dixie harrow, or Ely chain to bury seeds in Tooele County, Utah (Gunnell and Summers 2016). While broadcast seeding, drill seeding, surface compact seeding, or browse interseeding can be used to seed arrowleaf balsamroot (Monsen and Stevens 2004), covering seeds to protect them from rodent predation is critical (Shaw and Monsen 1983; Tilley et al. 2012).
Although earlier studies suggested shallower seeding depths (Wasser 1982; Monsen and Stevens 2004; Tilley et al. 2012), more recent observations indicate seeds should be planted at least 1 in (2.5 cm) deep (S. Monsen, USFS retired, personal communication, March 2018). Wasser (1982) suggested seeding at 0.5-in (1.3 cm) depths on medium-textured soils or moist sites and at 1 in (2.5 cm) on coarse-textured soils or dry sites. Monsen (USFS retired, personal communication, March 2018) suggests seeding at 1-in (2.5 cm) depths regardless of favorable soil or moisture conditions.
Seeding should occur in fall or late fall to allow for natural seed stratification. Although emergence can be uneven and continue for 2 to 3 years after fall seeding (Stevens and Monsen 2004), uniform germination and establishment are possible when good quality seed and appropriate seeding techniques are used (S. Monsen, USFS retired, personal communication, March 2018). Use of cool-moist stratified seed can improve emergence on sites where 3 months of continuous snow cover is not assured or to allow for early spring seeding (though not recommended) (Wasser 1982). In experimental testing, seed germination was good at temperatures of 32 to 41 ºF (0-5 ºC) but was reduced at lower temperatures, which suggested successful germination may be restricted to the snow-litter interface on sites with continuous snow cover for at least 3 months (Young and Evans 1979).
In field experiments snow cover did not impact germination but might have limited establishment (Kitchen and Monsen 1996). Regardless of the date of burial (June or October), 99% of bagged and protected seeds germinated at a site east of Boise, Idaho. This 3,180-ft (970 m) site received limited snow and the seed zone was frozen much of the winter. For unbagged and protected seeds, dry spring weather at the Idaho site resulted in early crusting of the soil surface and prevented emergence, which was less than 5% and restricted to cracks in the soil crust. Germination was 89% for seeds at a 4,920-ft (1,500 m) site east of Springville, Utah, which was relatively wet because of periodic winter rains. Seedling emergence was 49 to 69% at the Utah site for two seed accessions. Differences in germination among collections were not significantly correlated with climatic conditions of the seed collection site (Kitchen and Monsen 1996).
In field cultivation trials, use of a protective fabric resulted in some increased emergence of arrowleaf balsamroot (Jones and Whittaker 2011). A light-weight, permeable fabric increased heat and improved moisture retention during the germination period. At four sites, three in the Great Basin and one on the Colorado Plateau, percent germination averaged 5% with the fabric and less than 2% without the fabric. Benefits of the fabric were greatest at the driest sites. Use of the fabric also increased the cover of cheatgrass (Bromus tectorum) on sites where it was already present (Jones and Whittaker 2011).
Landeen and others (2021) found that arrowleaf balsamroot emergence and establishment was improved when row cover (N-sulate) (DeWitt Co, Sikeston, MO) was used to establish islands of native forbs in field experiments, but establishment and persistence were low overall. The study was conducted at four sites in Utah (three in Wyoming big sagebrush [Ecoregions 13 and 19] and one in mountain big sagebrush [Ecoregion 20]). Arrowleaf balsamroot emergence was often greater with row cover and density of plants was significantly greater (P < 0.05) on covered than uncovered plots in the fifth post-seeding year, but established plants remained immature and did not flower within the 5-year study period. Arrowleaf balsamroot was seeded in a mix with many other forbs at a rate of 3.9 PLS/ft² (41.9 PLS/m²), which is higher than typical. Sites were cleared of vegetation using a Dixie Harrow, seed was broadcast using a hand-crank seeder, and a Brillion imprinter and lightweight chain were used to incorporate the seed into the soil. Across all sites, arrowleaf balsamroot emergence was greater in covered than noncovered plots (P = 0.04). Plants persisted through the second year on all sites, but densities dropped substantially in the second year (Table 6). In control plots (unseeded with and without row cover), undesirable species increased with row cover treatments suggesting that increases in competition could accompany row cover treatments. Seed source may have also been a reason for limited establishment and persistence in this study, since adaptation to the site was not considered (Landeen et al. 2021)
Table 6. Mean density (plants/m²) of arrowleaf balsamroot with and without row cover (N-sulate) treatments following seeding at four sites in Utah (Landeen et al. 2021).
Year | ARTRW (Ecoregion 19) Fountain Green |
ARTRV (Ecoregion 20) Gordon Creek |
ARTRW (Ecoregion 13) Hatch Ranch |
ARTRW (Ecoregion 13) Lookout Pass |
All Sites | |||||
C | NC | C | NC | C | NC | C | NC | C | NC | |
1 | 2.48 | 1.46 | 2.28 | 1.76 | 3.37 | 0.89 | 0.99 | 0.65 | 2.28 | 1.19 |
2 | 0.15 | 0.12 | 1.01 | 0.60 | 1.43 | 0.43 | 0.29 | 0.25 | 0.72 | 0.35 |
5 | 0.03 | 0 | 0.53 | 0.43 | 5.80 | 1.63 | 0.43 | 0.13 | 1.70 | 0.55 |
Bold values within a site show significantly different plant densities between covered and noncovered plots (P < 0.001). C = covered, NC = not covered. ARTRW = Artemisia tridentata subsp. wyomingensis, ARTRV = A. t. subsp. vaseyana.
Stand management. Newly seeded areas need protection from grazing for many years (Stevens 2004b). Seedlings grow slowly, and even under best growing conditions, plants require 3 to 5 years to reach reproductive maturity (Stevens et al. 1996).
Studies indicate that arrowleaf balsamroot does not tolerate competition with spotted knapweed (Centaurea stoebe) and is sensitive to broadcast spraying of picloram to control spotted knapweed. In Palouse Prairie vegetation in western Montana, cover of arrowleaf balsamroot was 6.8% when spotted knapweed cover was less than 10% and less than 0.5% when spotted knapweed cover was 10 to 100% (Ridenour and Callaway 2001). In a rough fescue (Festuca campestris)-bluebunch wheatgrass natural area near Missoula, Montana, picloram herbicide applications in the fall did not affect leaf density but did impact reproduction of arrowleaf balsamroot. On sprayed plots, flowering was just 33% and recruitment 5% of that on unsprayed plots. Reductions in flowering and recruitment persisted at least 4 years (Crone et al. 2009). Ortega and Pearson (2010) found both cover and fecundity of arrowleaf balsamroot were reduced by as much as 60% with fall broadcast spraying of picloram in spotted knapweed–invaded bunchgrass communities in western Montana. Selective removal of spotted knapweed by clipping increased the abundance of arrowleaf balsamroot seedlings. At these sites, arrowleaf balsamroot inflorescence number and cover were negatively correlated (P < 0.001) with spotted knapweed cover (Ortega and Pearson 2010).
Acknowledgements
Funding for Western Forbs: Biology, Ecology, and Use in Restoration was provided by the USDI BLM Great Basin Native Plant Materials Ecoregional Program through the Great Basin Fire Science Exchange. Great thanks to the chapter reviewers: Steven Monsen, USDA FS (deceased), and Matt Fisk, USGS.
This research was supported in part by the USDA Forest Service, Rocky Mountain Research Station. The findings and conclusions in this publication are those of the authors and should not be construed to represent any official USDA or U.S. Government determination or policy.
Literature Cited
Amsberry, L.K.; Maron, J.L. 2006. Effects of herbivore identity on plant fecundity. Plant Ecology. 187(1): 39-48.
Association of Official Seed Analysts [AOSA]. 2010. AOSA/SCST Tetrazolium testing handbook. Contribution No. 29. Lincoln, NE: Association of Official Seed Analysts.
Association of Official Seed Analysts [AOSA]. 2023. AOSA rules for testing seeds. Vol. 1. Principles and procedures. Washington, DC: Association of Seed Analysts.
Austin, D.D.; Urness, P.J. 1998. Vegetal change on a northern Utah foothill range in the absence of livestock grazing between 1948 and 1982. Great Basin Naturalist. 58(2): 188-191.
Barner, J. 2009a. Propagation protocol for production of propagules (seeds, cuttings, poles, etc.) Balsamorhiza sagittata (Pursh) Nutt. seeds. Native Plant Network. U.S. Department of Agriculture, Forest Service, National Center for Reforestation, Nurseries, and Genetic Resources. http://npn.rngr.net/propagation/protocols [Accessed 2017 October 2].
Barner, J. 2009b. Propagation protocol for production of propagules (seeds, cuttings, poles, etc.) Balsamorhiza sagittata (Pursh) Nutt. seeds (2.1 pound lot). Native Plant Network. U.S. Department of Agriculture, Forest Service, National Center for Reforestation, Nurseries, and Genetic Resources. http://npn.rngr.net/propagation/protocols [Accessed 2017 October 2].
Basey, A.C.; Fant, J.B.; Kramer, A.T. 2015. Producing native plant materials for restoration: 10 rules to collect and maintain genetic diversity. Native Plants Journal. 16(1): 37-53.
Beck, J.L.; Peek, J.M. 2005a. Diet composition, forage selection, and potential for forage competition among elk, deer, and livestock on aspen-sagebrush summer range. Rangeland Ecology and Management. 58(2): 135-147.
Beck, J.L.; Peek, J.M. 2005b. Great Basin summer range forage quality: Do plants nutrients meet elk requirements? Western North American Naturalist. 65(4): 516-527.
Belcher, E. 1985. Handbook on seeds of browse-shrubs and forbs. Tech. Pub. No. R8-TP8. Atlanta, GA: U.S. Department of Agriculture, Forest Service, Southern Region. 246 p.
Best, T.L. 1993. Tamias ruficaudus. Mammalian Species. 452: 1-7.
BFI Native Seeds. 2017. Moses Lake, WA. http://www.bfinativeseeds.com/index.aspx [Accessed 2018 February 2]
Blaisdell, J.P. 1958. Seasonal development and yield of native plants on the Upper Snake River Plains of Idaho and their relation to climatic factors, especially precipitation and temperature. Tech. Bull. 1190. Washington, DC: U.S. Department of Agriculture. 68 p.
Blaisdell, J.P.; Pechanec, J.F. 1949. Effects of herbage removal at various dates on vigour of bluebunch wheatgrass and arrowleaf balsamroot. Ecology. 30(3): 298-305.
Blaisdell, J.P.; Wiese, A.C.; Hodgson, C.W. 1952. Variations in chemical composition of bluebunch wheatgrass, arrowleaf balsamroot, and associated range plants. Journal of Range Management. 5(5): 346-353.
Bloom, T.; Riginos, C.; O’Leary, D. 2018. For everything there was a season: Phenological shifts in the Tetons. University of Wyoming National Park Service Research Station annual report. 41: 11-35.
Bork, E.W.; West, N.E.; Walker, J.W. 1998. Cover components on long-term seasonal sheep grazing treatments in three-tip sagebrush steppe. Journal of Range Management. 51(3): 293-300.
Bowen, P.; Chambers, K.; Turner, J.; Keller, P.C. 2006. Propagation protocol for production of container (plug) Baslamorhiza sagittata (Pursh) Nutt. plants. Native Plant Network. U.S. Department of Agriculture, Forest Service, National Center for Reforestation, Nurseries, and Genetic Resources. http://npn.rngr.net/propagation/protocols [Accessed 2017 October 2].
Bower, A.D.; St. Clair, J.B.; Erickson, V. 2014. Generalized provisional seed zones for native plants. Ecological Applications. 24(5): 913-919.
Bradley, A.F.; Fischer, W.C.; Noste, N.V. 1992. Fire ecology of the forest habitat types of eastern Idaho and western Wyoming. Gen. Tech. Rep. INT-GTR-290. Ogden, UT: U.S. Department of Agriculture, Forest Service, Intermountain Research Station. 92 p.
Brown, A.; Allen, P.S. 2023. Elevation impact on seed germination requirements for two Asteraceae species. Native Plant Journal. 24(1): 45-53.
Brown, D.E. 1982. Great Basin montane scrubland. In: Brown, D.E., ed. Biotic communities of the American Southwest-United States and Mexico. Desert Plants. 4(1-4): 83-84.
Bruckart, W.L.; Eskandari, F.M.; Berner, D.K.; Aime, M.C. 2012. Comparison of Puccinia acroptili from Eurasia and the USA. Botany. 90(6): 465-471.
Buechner, H.K. 1960. The bighorn sheep in the United States, its past, present, and future. Wildlife Monographs. 4: 3-174.
Bujak, C.M.; Dougher, T.A.O. 2020. Fertilizer and container requirements of Balsamorhiza sagittata and Phacelia hastata var. hastata under greenhouse production. Native Plants Journal. 21(1): 15-25.
Bunting, S.C. 1987. Use of prescribed burning in juniper and pinyon-juniper woodlands. Res. Pap. INT-219. Ogden, UT: U.S. Department of Agriculture, Forest Service, Intermountain Research Station. 11 p.
Burrell, G.C. 1982. Winter diets of mule deer in relation to bitterbrush abundance. Journal of Range Management. 35(4): 508-510.
Camp, P.; Sanderson, J. 2007. Seed collection, propagation and reintroduction of native wildflowers in the Columbia Basin. Wenatchee, WA: U.S. Department of the Interior, Bureau of Land Management, Wenatchee Field Office. 32 p.
Cane, J.H. 2005. Pollination needs of arrowleaf balsamroot, Balsamorhiza sagittata (Heliantheae : Asteraceae). Western North American Naturalist. 65(3): 359-364.
Cane, J.H. 2011. Specialist Osmia bees forage indiscriminately among hybridizing Balsamorhiza floral hosts. Oecologia. 167(1): 107-116.
Cane, J.H. 2012. Pollination and breeding biology studies. In: Shaw, N.; Pellant, M., eds. Great Basin Native Plant Project: 2011 Progress Report. Boise, ID: U.S. Department of Agriculture, Forest Service, Rocky Mountain Research Station: 173-176.
Cane, J.H.; Love, B. 2016. Floral guilds of bees in sagebrush steppe: Comparing bee usage of wildflowers available for postfire restoration. Natural Areas Journal. 36(4): 377-391.
Chamberlin, R.V. 1911. The ethno-botany of the Gosiute Indians. American Anthropological Association. 63(1): 329-405.
Chambers, K.J.; Bowen, P.; Turner, N.J.; Keller, P.C. 2006. Ethylene improves germination of arrow-leaved balsamroot seeds. Native Plants. 7(2): 109-112.
Chambers, K.J.; Small, E.; Catling, P.M.; Turner, N.J.; Bowen, P.; Keller, C.P. 2002. Poorly known economic plants of Canada-35: Arrow-leaved balsamroot Balsamorhiza sagittata (Pursh.) Nutt. Canadian Botanical Association Bulletin. 35(4): 40-45.
Churchwell, R.; Ratti, J.T.; Edelmann, F. 2004. Comparison of fall and winter food habits for sympatric chukar and gray partridge in Hells Canyon of Idaho and Oregon. Northwest Science. 78(1): 42-47.
Clements, D.R.; Krannitz, P.G.; Gillespie, S.M. 2007. Seed bank responses to grazing history by invasive and native plant species in a semi-desert shrub-steppe environment. Northwest Science. 81(1): 37-49.
Consortium of Pacific Northwest Herbaria [CPNWH]. 2017. Seattle, WA: University of Washington Herbarium, Burke Museum of Natural History and Culture. http://www.pnwherbaria.org/index.php
Cook, C.W. 1954. Common use of summer range by sheep and cattle. Journal of Range Management. 7(1): 10-13.
Craddock, G.W.; Fording, C.L. 1938. The influence of climate and grazing on spring-fall sheep range in southern Idaho. Tech. Bull. 600. Washington, D.C.: U.S. Department of Agriculture, Forest Service. 43 p.
Crone, E.E.; Marler, M.; Pearson, D.E. 2009. Non-target effects of broadleaf herbicide on a native perennial forb: A demographic framework for assessing and minimizing impacts. Journal of Applied Ecology. 46(3): 673-682.
Cronquist, A.; Holmgren, A.H.; Holmgren, N.H.; Reveal, J.L.; Holmgren, P.K. 1994. Intermountain flora: Vascular plants of the Intermountain West, U.S.A. Volume 5: Asterales. Bronx, NY: The New York Botanic Garden. 496 p.
Day, T.; Wright, R.G. 1985. The vegetation types of Craters of the Moon National Monument. Bull. 38. Moscow, ID: University of Idaho, College of Forestry, Wildlife, and Range Sciences, Forest, Wildlife and Range Experiment Station. 6 p.
Devoe, J.D.; Proffitt, K.M.; Mitchell, M.S.; Jourdonnais, C.S.; Barker, K.J. 2019. Elk forage and risk tradeoffs during the fall archery season. The Journal of Wildlife Management. 83(4): 801-816.
Eldredge, E.; Novak-Echenique, P.; Heater, T.; Mulder, A.; Jasmine, J. 2013. Plants for pollinator habitat in Nevada. Tech. Note NV 57. Reno, NV: U.S. Department of Agriculture, Natural Resources Conservation Service. 65 p.
Elliott, C.L.; Flinders, J.T. 1984. Plant nutrient levels on two summer ranges in the River of No Return Wilderness Area, Idaho, USA. The Great Basin Naturalist. 44(4): 621-626.
Elliott, C.L.; Flinders, J.T. 1985. Food habits of the Columbian ground squirrel, Spermophilus columbianus, in southcentral Idaho. Canadian Field-Naturalist. 99(3): 327-330.
European Native Seed Conservation Network [ENSCONET]. 2009. ENSCONET seed collecting manual for wild species. Edition 1: 32 p.
Evans, R.A.; Easi, D.A.; Book, D.N.; Young, J.A. 1982. Quadratic response surface analysis of seed-germination trials. Weed Science. 30(4): 411-416.
Everett, R.L.; Meeuwig, R.O.; Stevens, R. 1978. Deer mouse preference for seed of commonly planted species, indigenous weed seed, and sacrifice foods. Journal of Range Management. 31(1): 70-73.
Farr, D.F.; Rossman, A.Y. 2023. Fungal databases, U.S. National Fungus Collections. U.S. Department of Agriculture, Agricultural Research Service. https://fungi.ars.usda.gov/
Franklin, J.F.; Dyrness, C.T. 1973. Natural vegetation of Oregon and Washington. Gen. Tech. Rep. PNW-8. Portland, OR: U.S. Department of Agriculture, Forest Service, Pacific Northwest Forest and Range Experiment Station. 452 p.
Gaines, W.L.; Lyons, A.L.; Weaver, K.; Sprague, A. 2011. Monitoring the short-term effects of prescribed fire on an endemic mollusk in the dry forest of the eastern Cascades, Washington, USA. Forest Ecology and Management. 261(8): 1460-1465.
Garrison, G.A.; Bjusgstad, A.J.; Duncan, D.A.; Lewis, M.E.; Smith, D.R. 1977. Vegetation and environmental features of forest and range ecosystems. Agric. Handb. 475. Washington, D.C.: U.S. Department of Agriculture, Forest Service. 68 p.
Gibson, E.H. 1918. The genus Corythucha Stal (Tingidae: Heteroptera). Transactions of the American Entomological Society. 44(1): 69-104.
Gokbulak, F. 2002. Effect of American bison (Bison bison L.) on the recovery and germinability of seeds of range forage species. Grass and Forage Science. 57(4): 395-400.
Goodey, J.B. 1948. The galls caused by Anguillulina balsamophila (Thorne) Goodey on the leaves of Wyethia amplexicaulis Nutt. and Balsamorhiza sagittata Nutt. Journal of Helminthology. 22(2): 109-116.
Gruell, G.E.; Schmidt, W.C.; Arno, S.F.; Reich, W.J. 1982. Seventy years of vegetative change in a managed ponderosa pine forest in western Montana-Implications for resource management. Gen. Tech. Rep. INT-130. Ogden, UT: U.S. Department of Agriculture, Forest Service, Intermountain Forest and Range Experiment Station. 42 p.
Gucker, C.L.; Bunting, S.C. 2011. Canyon grassland vegetation changes following fire in northern Idaho. Western North American Naturalist. 71(1): 97-105.
Gunnell, K.; Summers, D. 2016. Native forb increase and research at the Great Basin Research Center. In: Kilkenny, F.; Edwards, F.; Malcomb, A., eds. Great Basin Native Plant Project: 2015 Progress Report. Boise, ID: U.S. Department of Agriculture, Forest Service, Rocky Mountain Research Station: 96-108.
Habeck, J.R. 1976. Forest, fuels, and fire in the Selway-Bitterroot Wilderness, Idaho. In: Proceedings-Tall Timbers Fire Ecology Conference. 1974 October 8-10; Missoula, MT. Tall Timbers Research Station. 14: 305-353.
Hanson, W.R. 1951. Condition classes on mountain range in southwestern Alberta. Journal of Range Management. 4(3): 165-170.
Harniss, R.O.; Price, D.A.; Tomlin, D.C. 1975. Number of fistula samples needed for determination of sheep diet on sagebrush-grass range. Journal of Range Management. 28(5): 417-419.
Harniss, R.O.; Wright, H.A. 1982. Summer grazing of sagebrush-grass range by sheep. Journal of Range Management. 35(1): 13-17.
Hickman, J.C., ed. 1993. The Jepson manual: Higher plants of California. Berkeley, CA: University of California Press. 1400 p.
Hitchcock, C.L.; Cronquist, A. 2018. Flora of the Pacific Northwest: An illustrated manual. Second Ed. Giblin, D.E.; Legler, B.S.; Zika, P.F.; Olmstead, R.G., eds. Seattle, WA: University of Washington Press. 882 p.
Holechek, J.L.; Vavra, M.; Skovlin, J.; Krueger, W.C. 1982. Cattle diets in the Blue Mountains of Oregon, I. Grasslands. Journal of Range Management. 35(1): 109-112.
Horton, H. 1989. Interagency forage and conservation planting guide for Utah. AG-433. Logan, UT: Utah State University Extension. 84 p.
Hoskins, L.W.; Dalke, P.D. 1955. Winter browse on the Pocatello big game range in southeastern Idaho. Journal of Wildlife Management. 19(2): 215-225.
Houston, K.E.; Hartung, W.J.; Hartung, C.J. 2001. A field guide for forest indicator plants, sensitive plants, and noxious weeds of the Shoshone National Forest, Wyoming. Gen. Tech. Rep. RMRS-GTR-84. Ogden, UT: U.S. Department of Agriculture, Forest Service, Rocky Mountain Research Station. 184 p.
Jensen, C.H.; Smith, A.D.; Scotter, G.W. 1972. Guidelines for grazing sheep on rangelands used by big game in winter. Journal of Range Management. 25(5): 346-352.
Johnson, C.G. 1998. Vegetation response after wildfires in national forests of northeastern Oregon. R6-NR-ECOL-TP-06-98. Portland, OR: U.S. Department of Agriculture, Forest Service, Pacific Northwest Region. 128 p.
Johnson, C.G.; Swanson, D.K. 2005. Bunchgrass plant communities of the Blue and Ochoco Mountains: A guide for managers. Gen. Tech. Rep. PNW-GTR-641. Portland, OR: U.S. Department of Agriculture, Forest Service, Pacific Northwest Research Station. 119 p.
Johnston, A. 1970. Blackfoot Indian utilization of the flora of the northwestern Great Plains. Economic Botany. 24(3): 301-324.
Jones, C.; Whittaker, A. 2011. Native plant material development and cultivation trials for native Great Basin forbs and grasses. In: Shaw, N.; Pellant, M., eds. Great Basin Native Plant Selection and Increase Project 2010 Progress Report. Boise, ID: U.S. Department of Agriculture, Forest Service, Rocky Mountain Research Station: 57-63.
Jorgensen, K.R.; Stevens, R. 2004a. Seed collection, cleaning, and storage. In: Monsen, S.B.; Stevens, R.; Shaw, N.L., comps. Restoring western ranges and wildlands, vol. 3. Gen. Tech. Rep. RMRS-GTR-136-vol-3. Fort Collins, CO: U.S. Department of Agriculture, Forest Service, Rocky Mountain Research Station: 699-716.
Jorgensen, K.R.; Stevens, R. 2004b. Seed germination. In: Monsen, S.B.; Stevens, R.; Shaw, N.L., comps. Restoring western ranges and wildlands, vol. 3. Gen. Tech. Rep. RMRS-GTR-136-vol-3. Fort Collins, CO: U.S. Department of Agriculture, Forest Service, Rocky Mountain Research Station: 723-732.
Kasworm, W.F.; Irby, L.R.; Ihsle Pac, H.B. 1984. Diets of ungulates using winter ranges in northcentral Montana. Journal of Range Management. 37(1): 67-71.
Kilkenny, F.; Ott, J.; Barga, S.; Irwin, J.; Rizkowsky, K.; Campbell, N.; Richardson, B.; Milano, E.; Gucker, C. 2023. Great Basin Native Plant Project: 2022 Progress Report. Boise, ID: U.S. Department of Agriculture, Rocky Mountain Research Station. 53 p.
Kirol, C.P.; Beck, J.L.; Dinkins, J.B.; Conover, M.R. 2012. Microhabitat selection for nesting and brood-rearing by the greater sage-grouse in xeric big sagebrush. The Condor. 114(1): 75-89.
Kitchen, S.G. 1994. Perennial forb life-history strategies on semiarid rangelands: Implications for revegetation. In: Monsen, S.B.; Kitchen, S.G., comps. Proceedings – Ecology and management of annual rangelands; 1992 May 18-22; Boise, ID. Gen. Tech. Rep INT-GTR-313. Ogden, UT: U.S. Department of Agriculture, Forest Service, Intermountain Research Station: 342-346.
Kitchen, S.G.; Monsen, S.B. 1996. Arrowleaf balsamroot (Balsamorhiza sagittata) seed germination and establishment success (Utah). Restoration and Management Notes. 14(2): 180-181.
Kitchen, S.G.; Stevens, R.; Wilson, G.R. 1998. Addition of Balsamorhiza sagittata, arrowleaf balsamroot to the rules. The Seed Technologist Newsletter. 72(1): Proposal #55.
Klebenow, D.A. 1969. Sage grouse nesting and brood habitat in Idaho. The Journal of Wildlife Management. 33(3): 649-662.
Koniak, S. 1985. Succession in pinyon-juniper woodlands following wildfire in the Great Basin. Great Basin Naturalist. 45(3): 556-566.
Kruckeberg, A.R.; Chalker-Scott, L.; Olmstead, R.G. 2019. Native ornamental herbaceous perennials. In: Gardening with native plants of the Pacific Northwest. 3rd ed. Seattle, WA: University of Washington Press: 152-311.
Kuchler, A.W. 1964. Manual to accompany the map of potential vegetation of the conterminous United States. New York, NY: American Geographical Society Special Publication 36: 152.
Kufeld, R.C. 1973. Foods eaten by the Rocky Mountain elk. Journal of Range Management. 26(2): 106-113.
Kufeld, R.C.; Wallmo, O.C.; Feddema, C. 1973. Foods of the Rocky Mountain mule deer. Res. Pap. RM-111. Fort Collins, CO: U.S. Department of Agriculture, Forest Service, Rocky Mountain Forest and Range Experiment Station. 31 p.
Kulmatiski, A. 2006. Exotic plants establish persistent communities. Plant Ecology. 187(2): 261-275.
Kuntz, D.E. 1982. Plant response following spring burning in an Artemisia tridentata subsp. vaseyana/Festuca idahoensis habitat type. Moscow, ID: University of Idaho. Thesis. 73 p.
Lady Bird Johnson Wildflower Center [LBJWC]. 2017. Balsamorhiza sagittata (Pursh) Nutt. Native Plant Database. Austin, TX: Lady Bird Johnson Wildflower Center. https://www.wildflower.org/plants-main [Accessed 2017 October 2].
Landeen, M.; Jones, C.; Jensen, S.; Whittaker, A.; Summers, D.D.; Eggett, D.; Petersen, S.L. 2021. Establishing seed islands for native forb species on rangelands using N-Sulate ground cover fabric. Native Plants Journal. 22(1): 51-63.
Lavelle, D.A. 1986. Use and preference of spotted knapweed (Centaurea maculosa) by elk (Cervus elaphus) and mule deer (Odocoileus hemionus) on two winter ranges in western Montana. Missoula, MT: University of Montana. Thesis. 72 p.
Laycock, W.A. 1967. How heavy grazing and protection affect sagebrush-grass ranges. Journal of Range Management. 20(4): 206-213.
Lindbloom, A.J.; Reese, K.P.; Zager, P. 2003. Nesting and brood-rearing characteristics of chukars in west central Idaho. Western North American Naturalist. 63(4): 429-439.
Lovaas, A.L. 1958. Mule deer food habits and range use, Little Belt Mountains, Montana. Journal of Wildlife Management. 22(3): 275-283.
Major, J.; Rejmanek, M. 1992. Amelanchier alnifolia vegetation in eastern Idaho, USA and its environmental relationships. Vegetatio. 98(2): 141-156.
Marks, J.S.; Marks, V.S. 1987. Habitat selection by Columbian sharp-tailed grouse in west-central Idaho. Boise, ID: U.S. Department of the Interior, Bureau of Land Management. 115 p.
Maron, J.L.; Hajek, K.L.; Hahn, P.G.; Pearson, D.E. 2019. Seedling recruitment correlates with seed input across seed sizes. Ecology. 100(12): 1-11.
Marquiss, R.; Lang, R. 1959. Vegetational composition and ground cover of two natural relict areas and their associated grazed areas in the Red Desert of Wyoming. Journal of Range Management. 12(3): 104-109.
Matsuura, H.; Saxena, G.; Farmer, S.W.; Hancock, R.E.W.; Towers, G.H.N. 1996. An antibacterial thiophene from Balsamorhiza sagittata. Planta Medica. 62(1): 65-66.
McCombs, A.L.; Debinski, D.; Reinhardt, K.; Germino, M.J.; Caragea, P. 2022. Warming temperatures affect meadow-wide nectar resources, with implications for plant-pollinator communities. Ecosphere. 13(7 C7 – e4162).
McConnell, B.R.; Smith, J.G. 1970. Response of understory vegetation to ponderosa pine thinning in eastern Washington. Journal of Range Management. 23(3): 208-212.
McCutcheon, A.R.; Ellis, S.M.; Hancock, R.E.W.; Towers, G.H.N. 1994. Antifungal screening of medicinal plants of British Columbian native peoples. 44(3): 157-169.
Merrill, E.H.; Henry F.M.; Peek, J.M. 1980. Effects of a fall wildfire on herbaceous vegetation on xeric sites in the Selway-Bitterroot Wilderness, Idaho. Journal of Range Management. 33(5): 363-367.
Metlen, K.L.; Fiedler, C.E.; Youngblood, A. 2004. Understory response to fuel reduction treatments in the Blue Mountains of northeastern Oregon. Northwest Science. 78(3): 175-185.
Miller, R.F.; Chambers, J.C.; Pyke, D.A.; Pierson, F.B.; Williams, C.J. 2013. A review of fire effects on vegetation and soils in the Great Basin Region: Response and ecological site characteristics. Gen. Tech. Rep. RMRS-GTR-308. Fort Collins, CO: U.S. Department of Agriculture, Forest Service, Rocky Mountain Research Station. 126 p.
Moerman, D. 2003. Native American ethnobotany: A database of foods, drugs, dyes, and fibers of Native American peoples, derived from plants. Dearborn, MI: University of Michigan. http://naeb.brit.org
Mogensen, S.H.; Allen, P.S.; Meyer, S.E. 2001. Prechill temperature and duration are important in determining seed quality for 12 wildflowers. Seed Technology. 23(2): 145-150.
Monsen, S.B.; Shaw, N.L. 1983. Seeding antelope bitterbrush with grasses on south-central Idaho rangelands-A 39-year response. In: Tiedemann, A.R.; Johnson; K.L. Johnson, comps. Proceedings-Research and management of bitterbrush and cliffrose in western North America; 1982 April 13-15, Salt Lake City, UT. Gen. Tech. Rep. INT-152. Ogden, UT: U.S. Department of Agriculture, Forest Service, Intermountain Forest and Range Experiment Station: 126-136.
Monsen, S.B.; Stevens, R. 2004. Seedbed preparation and seeding practices. In: Monsen, S.B.; Stevens, R.; Shaw, N.L., comps. Restoring western ranges and wildlands, vol. 1. Gen. Tech. Rep. RMRS-GTR-136-vol-1. Fort Collins, CO: U.S. Department of Agriculture, Forest Service, Rocky Mountain Research Station: 121-154.
Monthony, A.S.; Baethke, K.; Erland, L.A.E.; Murch, S.J. 2020. Tools for conservation of Balsamorhiza deltoidea and Balsamorhiza sagittata: Karrikin and thidiazuron-induced growth. In Vitro Cellular & Developmental Biology – Plant. 56(3): 398-406.
Moore, A.J.; Bohs, L. 2003. An ITS Phylogeny of Balsamorhiza and Wyethia (Asteraceae: Heliantheae). American Journal of Botany. 90(11): 1653-1660.
Morris, M.S.; Schwartz, J.E. 1957. Mule deer and elk food habits on the National Bison Range. Journal of Wildlife Management. 21(2): 189-193.
Mueggler, W.F.; Harris, C.A. 1969. Some vegetation and soil characteristics of mountain grasslands in central Idaho. Ecology. 50(4): 671-678.
Mueggler, W.F.; Stewart, W.L. 1980. Grassland and shrubland habitat types of western Montana. Gen. Tech. Rep. INT-66. Ogden, UT: U.S. Department of Agriculture, Forest Service, Intermountain Forest and Range Experiment Station. 154 p.
Mullin, W.J.; Peacock, S.; Loewen, D.C.; Turner, N.J. 1997. Macronutrients content of yellow glacier lily and balsamroot; root vegetables used by indigenous peoples of northwestern North America. Food Research International. 30(10): 769-775.
Neely, E.E.; Barkworth, M.E. 1984. Vegetation on soils derived from dolomite and quartzite in the Bear River Range, Utah: A comparative study. Bulletin of the Torrey Botanical Club. 111(2): 179-192.
Nellis, C.H.; Ross, R.L. 1969. Changes in mule deer food habits associated with herd reduction. The Journal of Wildlife Management. 33(1): 191-195.
Ogle, D.; Tilley, D.; Cane, J.; St. John, L.; Fullen, K.; Stannard, M.; Pavek, P. 2011. Plants for pollinators in the Intermountain West. Plant Materials Tech. Note 2A. Boise, ID: U.S. Department of Agriculture, Natural Resources Conservation Service. 40 p.
Ogle, D.; Tilley, D.; Cane, J.; St. John, L.; Fullen, K.; Stannard, M.; Pavek, P. 2019. Plants for pollinators in the Intermountain West. Plant Materials Tech. Note 2A. Boise, ID: U.S. Department of Agriculture, Natural Resources Conservation Service. 40 p.
Omernik, J.M. 1987. Ecoregions of the conterminous United States. Map (scale 1:7,500,000). Annals of the Association of American Geographers. 77(1): 118-125.
Ortega, Y.K.; Pearson, D.E. 2010. Effects of picloram application on community dominants vary with initial levels of spotted knapweed (Centaurea stoebe) invasion. Invasive Plant Science and Management. 3(1): 70-80.
Ownbey, M.; Weber, W.A. 1943. Natural hybridization in the genus Balsamorhiza. American Journal of Botany. 30(3): 179-187.
Passey, H.B.; Hugie, V.K.; Williams, E.W.; Ball, D.E. 1982. Relationships between soil, plant community, and climate on rangelands of the Intermountain West. Tech. Bull. No. 1669. Washington, D.C. U.S. Department of Agriculture, Soil Conservation Service. 123 p.
Pavek, P.; Erhardt, B.; Heekin, T.; Old, R. 2012. Forb seedling identification guide for the Inland Northwest: Native, introduced, invasive, and noxious species. Pullman, WA: U.S. Department of Agriculture, Natural Resources Conservation Service, Pullman Plant Materials Center. 144 p.
Peacock, S.L. 2008. From complex to simple: Balsamroot, inulin, and the chemistry of traditional Interior Salish pit-cooking technology. Botany. 86(2): 116-128.
Pearson, D.E.; Callaway, R.M. 2008. Weed-biocontrol insects reduce native-plant recruitment through second-order apparent competition. Ecological Applications. 18(6): 1489-1500.
Pechanec, J.F.; Pickford, G.D.; Stewart, G. 1937. Effects of the 1934 drought on native vegetation of the upper Snake River Plains, Idaho. Ecology. 18(4): 490-505.
Peterson, S.L.; Stringham, T.K. 2009. Intercanopy community structure across a heterogeneous landscape in a western juniper-encroached ecosystem. Journal of Vegetation Science. 20(6): 1163-1175.
Powell, D.C.; Johnson, C.G., Jr.; Crowe, E.A.; Wells, A.; Swanson, D.K. 2007. Potential vegetation hierarchy for the Blue Mountains section of northeastern Oregon, southeastern Washington, and west-central Idaho. Gen. Tech. Rep. PNW-GTR-709. Portland, OR: U.S. Department of Agriculture, Forest Service, Pacific Northwest Research Station. 87 p.
Rehn, J.A.G.; Rehn, J.W.H. 1945. Studies of certain Cyrtacanthacridoid genera (Orthoptera: Acrididae). Part III. Transactions of the American Entomological Society. 71(1/2): 1-45.
Richardson, B.; Kilkenny, F.; St. Clair, B.; Stevenson-Molnar, N. 2020. Climate Smart Restoration Tool. https://climaterestorationtool.org/csrt/
Ridenour, W.M.; Callaway, R.M. 2001. The relative importance of allelopathy in interference: The effects of an invasive weed on a native bunchgrass. Oecologia. 126(3): 444-450.
Riegel, G.M.; Thornburgh, D.A.; Sawyer, J.O. 1990. Forest habitat types of the South Warner Mountains, Modoc County, California. Madrono. 37(2): 88-112.
Ripplinger, J.; Franklin, J.; Edwards, T.C. 2015. Legacy effects of no-analogue disturbances alter plant community diversity and composition in semi-arid sagebrush steppe. Journal of Vegetation Science. 26(5): 923-933.
Robinson, G.S.; Ackery, P.R.; Kitching, I.; Beccaloni, G.W.; Hernández, L.M. 2023. HOSTS: A Database of the World’s Lepidopteran Hostplants [Data set]. Natural History Museum. https://doi.org/10.5519/havt50xw
Rumsey, W.B. 1971. Range seedings versus climax vegetation on three sites in Idaho. Journal of Range Management. 24(6): 447-450.
Saab, V.A.; Marks, J.S. 1992. Summer habitat use by Columbian sharp-tailed grouse in western Idaho. Great Basin Naturalist. 52(2): 166-173.
Schallenberger, A.D. 1966. Food habits, range use and interspecific relationships of bighorn sheep in the Sun River area, west-central Montana. Bozeman, MT: Montana State University. Thesis. 44 p.
Schmidt, W.C.; Lotan, J.E. 1980. Phenology of common forest flora of the Northern Rockies-1928 to 1937. Res. Pap. INT-RP-259. Ogden, UT: U.S. Department of Agriculture, Forest Service, Intermountain Forest and Range Experiment Station. 20 p.
Schwartz, M.D. 1984. A revision of the black grass bug genus Irbisia reuter (Heteroptera: Miridae). Journal of the New York Entomological Society. 92(3): 193-306.
Schwartz, M.D.; Scudder, G.G.E. 2000. Miridae (Heteroptera) new to Canada, with some taxonomic changes. Journal of the New York Entomological Society. 108(3/4): 248-267.
SEINet – Regional Networks of North American Herbaria Steering Committee [SEINet]. 2017. SEINet Regional Networks of North American Herbaria. https://symbiota.org/seinet
Sharp, W.M. 1935. A critical study of certain Epappose genera of the Heliantheae-Verbesininae of the natural family Compositae. Annals of the Missouri Botanical Garden. 22(1): 51-152.
Shaw, N.L.; Monsen, S.B. 1983. Nonleguminous forbs for rangeland sites. In: Monsen S.B.; Shaw N.L., comps. Managing Intermountain rangelands-Improvement of range and wildlife habitats; 1981 September 15-17; Twin Falls, ID; 1982 June 22-24; Elko, NV. Gen. Tech. Rep. INT-157. Ogden, UT: U.S. Department of Agriculture, Forest Service, Intermountain Forest and Range Experiment Station: 123-131.
Sheley, R.L.; Half, M.L. 2006. Enhancing native forb establishment and persistence using a rich seed mixture. Restoration Ecology. 14(4): 627-635.
Shiflet, T.N. 1994. Rangeland cover types of the United States. Denver, CO: Society for Range Management. 152 p.
Shock, C.; Feibert, E.; Parris, C.; Saunders, L. 2012. Direct surface seeding strategies for establishment of Intermountain West native plants for seed production. In: Shock, C.C., ed. Malheur Experiment Station Annual Report 2011. OSU AES Ext/CrS141. Corvallis, OR: Oregon State University: 130-135.
Skinner, D. 2004. Propagation protocol for production of container (plug) Balsamorhiza sagittata (Pursh) Nutt. plants. Native Plant Network. U.S. Department of Agriculture, Forest Service, National Center for Reforestation, Nurseries, and Genetic Resources. http://npn.rngr.net/propagation/protocols [Accessed 2017 October 2].
Smith, A.D. 1949. Effects of mule deer and livestock upon a foothill range in northern Utah. The Journal of Wildlife Management. 13(4): 421-423.
Smith, A.D. 1953. Consumption of native forage species by captive mule deer during summer. Journal of Range Management. 6(1): 30-37.
Society for Ecological Restoration; International Network for Seed Based Restoration; Royal Botanic Gardens [SER, INSR, RBGK]. 2023. Seed Information Database (SID). Available from: https://ser-sid.org/
Steele, R.; Geier-Hayes, K. 1995. Major Douglas-fir habitat types of central Idaho: A summary of succession and management. Gen. Tech. Rep. INT-GTR-331. Ogden, UT: U.S. Department of Agriculture, Forest Service, Intermountain Research Station. 23 p.
Stevens, R. 1983. Species adapted for seeding mountain brush, big, black, and low sagebrush, and pinyon-juniper communities. In: Monsen S.B.; Shaw N.L., comps. Managing Intermountain rangelands-Improvement of range and wildlife habitats; 1981 September 15-17; Twin Falls, ID; 1982 June 22-24; Elko, NV. Gen. Tech. Rep. INT-157. U.S. Department of Agriculture, Forest Service, Intermountain Forest and Range Experiment Station: 78-82.
Stevens, R. 2004a. Establishing plants by transplanting and interseeding. In: Monsen, S.B.; Stevens, R.; Shaw, N.L., comps. Restoring western ranges and wildlands, vol. 3. Gen. Tech. Rep. RMRS-GTR-136-vol-3. Fort Collins, CO: U.S. Department of Agriculture, Forest Service, Rocky Mountain Research Station: 739-744.
Stevens, R. 2004b. Management of restored and revegetated sites. In: Monsen, S.B.; Stevens, R.; Shaw, N.L., comps. Restoring western ranges and wildlands, vol. 1. Gen. Tech. Rep. RMRS-GTR-136-vol-1. Fort Collins, CO: U.S. Department of Agriculture, Forest Service, Rocky Mountain Research Station: 193-198.
Stevens, R.; Jorgensen, K.R.; Davis, J.N. 1981. Viability of seed from thirty-two shrub and forb species through fifteen years of warehouse storage. Great Basin Naturalist. 41(3): 274-277.
Stevens, R.; Monsen, S.B. 2004. Forbs for seeding range and wildlife habitats. In: Monsen, S.B.; Stevens, R.; Shaw, N.L., comps. Restoring western ranges and wildlands, vol. 2. Gen. Tech. Rep. RMRS-GTR-136-vol-2. Fort Collins, CO: U.S. Department of Agriculture, Forest Service, Rocky Mountain Research Station: 425-466.
Stevens, R.J.; Jorgensen, K.R.; Young, A.S.; Monsen, S.B. 1996. Forb and shrub seed production guide for Utah. AG 501. Logan, UT: Utah State University Extension. 52 p.
Stubbendieck, J.; Hatch, S.L.; Hirsch, K.J. 1986. North American range plants. 3rd ed. Lincoln, NE: University of Nebraska Press. 465 p.
Taylor, R.J. 1992. Sagebrush country: A wildflower sanctuary. Missoula, MT: Mountain Press Publishing Company. 211 p.
Tilley, D.; St. John, L.; Shaw, N. 2012. Plant guide: Arrowleaf balsamroot (Balsamorhiza sagittata [Pursh] Nutt.). Aberdeen, ID: U.S. Department of Agriculture, Natural Resources Conservation Service, Aberdeen Plant Materials Center. 5 p.
Tisdale, E.W. 1947. The grasslands of the southern Interior of British Columbia. Ecology. 28(4): 346-382.
Treshow, M.; Harper, K. 1974. Longevity of perennial forbs and grasses. Oikos. 25(1): 93-96.
Turner, N.J.; Ignace, M.B.; Ignace, R. 2000. Traditional ecological knowledge and wisdom of aboriginal peoples in British Columbia. Ecological Applications. 10(5): 1275-1287.
USDA Forest Service [USDA FS] 1937. Range plant handbook. Washington, DC: U.S. Department of Agriculture, Forest Service. 816 p.
USDA Forest Service, Bend Seed Extractory [USDA FS BSE]. 2017. Nursery Management Information System Version 4.1.11. Local Source Report 34-Source Received. Bend, OR: U.S. Department of Agriculture, Forest Service, Bend Seed Extractory.
USDA Forest Service, Bend Seed Extractory [USDA FS BSE]. 2023. Nursery Management Information System Version 4.1.11. Local Source Report 34-Source Received. Bend, OR: U.S. Department of Agriculture, Forest Service Bend Seed Extractory.
USDA Forest Service, Great Basin Native Plant Project [USFS GBNPP]. 2014. Seed weight table calculations made in-house. Report on file. Boise, ID: U.S. Department of Agriculture, Forest Service, Boise Aquatic Sciences Laboratory. Available: https://www.fs.fed.us/rm/boise/research/shrub/Links/Seedweights.pdf
USDA Forest Service, Western Wildland Environmental Threat Assessment Center [USFS WWETAC]. 2024. TRM Seed Zone Applications. Prineville, OR: U.S. Department of Agriculture, Forest Service, Western Wildland Environmental Threat Assessment Center. Available: https://www.fs.usda.gov/wwetac/threat-map/TRMSeedZoneMapper.php
USDA Natural Resources Conservation Service [USDA NRCS]. 2000. Washington and Oregon guide for conservation seedings and plantings. Portland, OR: U.S. Department of Agriculture, Natural Resources Conservation Service. 126 p.
USDA Natural Resources Conservation Service [USDA NRCS]. 2024. The PLANTS Database. Greensboro, NC: U.S. Department of Agriculture, Natural Resources Conservation Service, National Plant Data Team. https://plants.usda.gov/home
USDI Bureau of Land Management, Seeds of Success [USDI BLM SOS]. 2017. Seeds of Success collection data. Washington, DC: U.S. Department of the Interior, Bureau of Land Management, Plant Conservation and Restoration Program.
USDI Bureau of Land Management, Seeds of Success [USDI BLM SOS]. 2023. Bureau of Land Management technical protocol for the collection, study, and conservation of seeds from native plant species for Seeds of Success. Washington, DC: U.S. Department of the Interior, Bureau of Land Management, Plant Conservation and Restoration Program. 45 p.
USDI Bureau of Land Management, Seeds of Success [USDI BLM SOS]. 2024. Seeds of Success collection data. Washington, DC: U.S. Department of the Interior, Bureau of Land Management, Plant Conservation and Restoration Program.
USDI Environmental Protection Agency [USDI EPA]. 2017. Ecoregions. Washington, DC: U.S. Department of the Interior, Environmental Protection Agency. https://www.epa.gov/eco-research/ecoregions
USDI Geological Survey [USDI USGS]. 2017. Biodiversity Information Serving Our Nation (BISON). U.S. Geological Survey. https://bison.usgs.gov/#home
Utah Crop Improvement Association [UCIA]. 2015. How to be a seed connoisseur. Logan, UT: UCIA, Utah Department of Agriculture and Food, Utah State University and Utah State Seed Laboratory. 16 p.
Vallentine, J.F. 1989. Range development and improvements. Third ed. San Diego, CA: Academic Press. 524 p.
Van Epps, G.A.; Stevens, R. 2004. Shrub and forb seed production. In: Monsen, S.B.; Stevens, R.; Shaw, N.L., comps. Restoring western ranges and wildlands, vol. 3. Gen. Tech. Rep. RMRS-GTR-136-vol-3. Fort Collins, CO: U.S. Department of Agriculture, Forest Service, Rocky Mountain Research Station: 717-722.
Wagner, G.D.; Peek, J.M. 2006. Bighorn sheep diet selection and forage quality in central Idaho. Northwest Science. 80(4): 246-258.
Wasser, C.H. 1982. Ecology and culture of selected species useful in revegetating disturbed lands in the West. FWS/OBS-82/56. Washington, D.C.: U.S. Department of the Interior, Fish and Wildlife Service, Office of Biological Services, Western Energy and Land Use Team. 347 p.
Weaver, J.E. 1915. A study of the root systems of prairie plants of southeastern Washington (Concluded). The Plant World. 18(10): 273-292.
Weaver, J.E. 1958. Classification of root systems of forbs of grassland and a consideration of their significance. Ecology. 39(3): 394-401.
Weber, W.A. 2006. 273. Balsamorhiza. In: Flora of North America Editorial Committee, ed. Flora of North America North of Mexico. Volume 21 Magnoliophyta: Asteridae, part 8: Asteraceae, part 3 Asterales, part 3 (Aster order). New York, NY: Oxford University Press: 91-98.
Weiner, N.I.; Strand, E.K.; Bunting, S.C.; Smith, A.M.S. 2016. Duff distribution influences fire severity and post-fire vegetation recovery in sagebrush steppe. Ecosystems. 19(7): 1196-1209.
Welsh, S.L.; Atwood, N.D.; Goodrich, S.; Higgins, L.C., eds. 2015. A Utah Flora. Fifth Edition, revised. Provo, UT: Brigham Young University. 990 p.
Wikeem, B.M.; Pitt, M.D. 1979. Interpreting diet preference of California bighorn sheep on native rangeland in south-central British Columbia. Rangelands. 1(5): 200-202.
Wikeem, B.M.; Pitt, M.D. 1991. Grazing effects and range trend assessment on California bighorn sheep range. Journal of Range Management. 44(5): 466-470.
Wilkins, B.T. 1957. Range use, food habits, and agricultural relationships of the mule deer, Bridger Mountains, Montana. The Journal of Wildlife Management. 21(2): 159-169.
Young, J.A.; Evans, R.A. 1978. Population dynamics after wildfires in sagebrush grasslands. Journal of Range Management. 31(4): 283-289.
Young, J.A.; Evans, R.A. 1979. Arrowleaf balsamroot and mules ear seed germination. Journal of Range Management. 32(1): 71-74.
Young, S.A.; Schrumpf, B.; Bouck, M.; Moore, M. 2020. How the Association of Official Seed Certifying Agencies (AOSCA) tracks wildland sourced seed and other plant propagating materials. Logan, UT: Utah State University, Utah Agricultural Experiment Station. 36 p.
Zschaechner, G.A. 1985. Studying rangeland fire effects: A case study in Nevada. In: Saunders, K.; Durham, J.; [and others], eds. Rangeland fire effects: A symposium. 1984 November 27-29; Boise, ID. Boise, ID: U.S. Department of the Interior, Bureau of Land Management, Idaho State Office: 66-84.
How to Cite
Gucker, Corey L.; Shaw, Nancy L. 2024. Arrowleaf balsamroot (Balsamorhiza sagittata). In: Gucker, C.L.; Shaw, N.L., eds. Western forbs: Biology, ecology, and use in restoration. Reno, NV: Great Basin Fire Science Exchange. Online: http://westernforbs.org