Authorship
Gucker, Corey; Shaw, Nancy
Publication Date
September 2023
Nomenclature
Basalt milkvetch (Astragalus filipes Torr. ex. A. Gray) belongs to the Fabaceae or pea family, the Galegeae tribe, and Cusickiani section (Barneby 1964; Sanderson 1991).
Family
Fabaceae – Pea family
Genus
Astragalus
Species
filipes
NRCS Plant Code
ASFI (USDA NRCS 2023).
Subtaxa
None
Synonyms
Astragalus filipes var. residuus Jeps., A. macgregorii (Rydb.) Tidestr., A. stenophyllus Torr. & A. Gray, and A. s. var. filipes Torr. ex A. Gray (ITIS 2023).
Common Names
Basalt milkvetch, Snakeriver plains milkvetch, threadsilk milkvetch, threadstalk milkvetch (Youtie and Miller 1986; Lambert 2005; ITIS 2023)
Chromosome Number
2n = 22, 24 (Spellenberg 1976; Welsh et al. 2016).
Hybridization
Zimmers et al. (2017) suspected gene flow between basalt milkvetch and Packard’s milkvetch (A. cusickii var. packardiae) where distributions overlap in southwestern Idaho and adjacent Oregon.
Distribution
Basalt milkvetch is one of the most common and widely dispersed Astragalus species (Barneby 1964; Johnson et al. 2008). It occurs in western North America from southern British Columbia to northern Mexico where annual precipitation ranges from 5 to 30 in (127-762 mm) (Tilley et al. 2017). Populations are somewhat isolated in southern British Columbia and disjunct and scattered in the mountains of interior southern California and northern Baja California (Barneby 1964). Populations are widespread and locally abundant in the Columbia Basin and Great Basin (Barneby 1989; Mansfield 2000), especially the Northern Basin and Range, Central Basin and Range, Blue Mountains, and Columbia Plateau Omernik Level III Ecoregions (Barneby 1964; Johnson et al. 2008). Basalt milkvetch occurs only in Box Elder County in Utah (Welsh et al. 2016).
Habitat And Plant Associations
Basalt milkvetch occupies dry hillsides, rolling plains, and valley floors in grassland, sagebrush (Artemisia spp.), pinyon-juniper (Pinus–Juniperus spp.), chaparral, and ponderosa pine (P. ponderosa) vegetation (Figs. 1 and 2) (Barneby 1964; Munz and Keck 1973; Pavek et al. 2012; Welsh et al. 2016; Tilley et al. 2017). In seven old-growth western juniper (J. occidentalis) sites in central Oregon, basalt milkvetch abundance increased with increasing elevation and slope and decreased with increasing sand content (Waichler et al. 2001). In the Owyhee Upland Province in southeastern Oregon, basalt milkvetch is characteristic of the big sagebrush/bluebunch wheatgrass (A. tridentata/Pseudoroegneria spicata) plant association (Culver 1964 cited in Franklin and Dyrness 1973). At the Idaho National Engineering Laboratory in southeastern Idaho, basalt milkvetch grew in native Wyoming big sagebrush (A. t. subsp. wyomingensis) and basin big sagebrush (A. t. subsp. tridentata). It was also one of the few species that established in nonnative crested wheatgrass (Agropyron cristatum) stands seeded more than 20 years earlier (Marlette and Anderson 1986).
Figure 1. Basalt milkvetch growing with sagebrush in Oregon. Photo: USDI Bureau of Land Management (BLM) OR010, Seeds of Success (SOS).
Figure 2. Basalt milkvetch growing in an early seral grassland in big sagebrush habitat in Oregon. Photo: USDI BLM OR030, SOS.
Elevation
Across its range, basalt milkvetch occurs at elevations of 250 to 8,000 ft (80-2,400 m) (Barneby 1964; Tilley et al. 2017), although populations are more common below 6,600 ft (2,000 m) (Barneby 1989). In California, basalt milkvetch grows in sagebrush scrub at elevations of 3,400 to 5,000 ft (1,000-1,500 m) (Munz and Keck 1973). On Steens Mountain, Nevada, it occurs at elevations below 5,600 ft (1,700 m) (Mansfield 2000). In Utah, it grows at elevations ranging from 5,200 to 6,000 ft (1,585-1,830 m) (Welsh et al. 2016).
Soils
Basalt milkvetch has low moisture requirements, tolerates low salinity (Eldredge et al. 2013), and is common on basalt-derived soils (Barneby 1964). Plants are also occasional on granitic or sedimentary bedrock soils (Barneby 1964). Soil texture is often sandy, loamy, or gravelly (Barneby 1964). In Nevada, a soil pH range of 5.8 to 7.3 was reported for basalt milkvetch (Eldredge et al. 2013). In the Blue and Ochoco Mountains in Oregon, basalt milkvetch was up to 100% constant in the ponderosa pine/antelope bitterbrush (Purshia tridentata)/Idaho fescue (Festuca idahoensis)-bluebunch wheatgrass plant association on basalt and rhyolite at 4,000 to 5,260 ft (1,200-1,600 m). Soils were 6 to 16 in (15-41 cm) deep loams over very to extremely gravelly or cobbly subsoils (Johnson and Swanson 2005). Basalt milkvetch was common in mountain sagebrush/Thurber’s needlegrass (A. t. subsp. vaseyana/Achnatherum thurberianum) communities in southeastern Oregon and northeastern California. This community was the driest of the mountain sagebrush communities visited and occurred on south slopes. It occupied loam, sandy loam, and fine loam soils that were 14 to 26 in (36-67 cm) deep over duripan (Miller et al. 2000).
Description
Basalt milkvetch is a slender to robust herbaceous perennial or subshrub from a thick, woody taproot and a superficially or shallowly buried branching root crown (Fig. 3) (Barneby 1964, 1989; Munz and Keck 1973; Pavek et al. 2012; Tilley et al. 2017). Plants produce many upright to ascending, slender, clumping stems up to 36 in (91 cm) tall (Barneby 1964; Pavek et al. 2012; Welsh et al. 2016; Tilley et al. 2017). Stems have few leaves and are purplish at the base (Barneby 1964; Munz and Keck 1973). Herbage varies from nearly glabrous to densely strigulose with straight, appressed, basifixed hairs (Barneby 1964, 1989; Munz and Keck 1973). Stem leaves are short petiolate with the uppermost subsessile (Barneby 1964). Leaves are alternate, 1 to 4.7 in (2.5-12 cm) long, and odd pinnately compound with 5 to 25 leaflets, although 9 to 19 leaflets are most common (Barneby 1964; Munz and Keck 1973; Pavek et al. 2012; Welsh et al. 2016). Leaflets are linear to narrowly elliptic or oblong, 0.1 to 1.2 in (3-30 mm) long, 0.5 to 5 mm wide, and often distantly scattered along the axis (Barneby 1964, 1989; Welsh et al. 2016). Leaflets are often truncate and retuse and glabrous or strigose on one or both sides (Barneby 1964; Munz and Keck 1973; Welsh et al. 2016). Stipules are dimorphic, the upper stipules are herbaceous, small, and free of the stem while the lower stipules are 2-5 mm long and connate with a papery sheath (Munz and Keck 1973; Barneby 1989).
Figure 3. Mature basalt milkvetch plant flowering in Oregon. Photo: USDI BLM OR010, SOS.
Inflorescences are loose axillary racemes 1.8 to 9.8 in (4.5-25 cm) long with 4 to 30 pea-like flowers that rise above the foliage (Fig. 4) (Barneby 1964; Munz and Keck 1973; Pavek et al. 2012; Welsh et al. 2016). Flower abundance decreases with plant age (Barneby 1989). Individual flowers are papilionaceous, spreading to declined or nodding, and 10 to 14.3 mm long with five white- or cream-colored petals (Barneby 1964, 1989; Mansfield 2000; Welsh et al. 2016). The banner is recurved about 50 to 85 degrees, 10 to 15.5 mm long, and 4.5 to 10 mm wide (Barneby 1964). Wings are 9 to 15 mm long, and the claws with slightly recurved blades are 3.5 to 6 mm long (Barneby 1964). Flower tubes are subcylindric to campanulate and strigulose with black and white hairs (Barneby 1989; Welsh et al. 2016). Stamens are diadelphous, and the style is glabrous (Welsh et al. 2016). Fruits are pendulous, glabrous, pods (Barneby 1964; Munz and Keck 1973; Mansfield 2000; Welsh et al. 2016). Pods are narrowly oblong to elliptic, 0.7 to 1.2 in (1.7-3 cm) long, 3 to 6.5 mm wide, and straight or nearly so (Fig. 5) (Barneby 1964, 1989; Munz and Keck 1973; Mansfield 2000; Welsh et al. 2016). They are tapered at both ends, strongly compressed, and unilocular (Barneby 1964; Munz and Keck 1973). Sutures are commonly parallel and brownish with 6 to 16 mm long stipes (Barneby 1964; Welsh et al. 2016). Pods contain 11 to 22 ovules (Barneby 1964, 1989; Welsh et al. 2016). Seeds are smooth and dull, pale to reddish brown and up to 3.1 mm long (Barneby 1964).
Figure 4. Terminal raceme inflorescences of a basalt milkvetch plant growing among grasses in Idaho. Photo: USDI BLM ID931, SOS.
Figure 5. Basalt milkvetch plant with fresh (green), older (straw color), and dropped (naked stem tips) pods. Photo: USDI BLM OR030, SOS.
Palouse milkvetch (Astragalus arrectus), Canada milkvetch (A. canadensis), cicer milkvetch (A. cicer), and hairy vetch (Vicia villosa) are lookalike species (Pavek et al. 2012).
Belowground Relationships And Interactions
Basalt milkvetch produces root nodules with nitrogen (N)-fixing bacteria (Bhattarai 2007; Johnson et al. 2008). Six rhizobial strains were isolated from the root nodules of basalt milkvetch in a greenhouse study and used to inoculate several basalt milkvetch accessions. Researchers identified one rhizobial strain that consistently exhibited exceptional N fixation across accessions. Plants inoculated with this rhizobium produced the greatest nodule weight, greatest number of nodules, and highest shoot N and total N pools. Total N was highly correlated with plant biomass (P < 0.001) suggesting plants more efficiently using N grew larger (Bhattarai 2007).
Reproduction
Basalt milkvetch reproduces sexually by seed, and seed production is increased when flowers are outcrossed through insect pollination (see below). Flowering occurs from May to July in the Intermountain West (Ogle et al. 2019).
In a common garden study, Bhattarai (2007) observed new shoots developing from lateral roots. Creeping roots were not consistently observed with any particular accession but did occur on several occasions (Bhattarai 2007).
Pollination
Basalt milkvetch requires pollinators for good seed set (Watrous and Cane 2011), Reproductive output was compared for four manual pollination treatments in a common garden in Logan, Utah (Fig. 6) (Watrous 2010; Watrous and Cane 2011). Manual pollination treatments were applied to 830 flowers on plants grown from seed collected from eight unique ecoregions (Omernik Level IV Ecoregions in WA, OR, NV, ID, and CA). Pollination treatments included autogamy where pollen was applied to the stigma of the same flower; geitonogamy where pollen was transferred between flowers on the same plant; xenogamy where pollen was transferred between different plants grown from seed collected at the same location; and distant xenogamy where pollen was transferred between different plants grown from seed collected from different ecoregions. Fruit and seed set were significantly greater with cross pollination (xenogamy) than with autogamy or geitonogamy (P <0.05). Autogamous pollination resulted in more empty pods than geitonogamous pollination (P = 0.024), which in turn resulted in more empty pods than xenogamous pollination (P = 0.001) (Fig. 6). Cross pollination (xenogamy) yielded nine times more seed than autogamy (93 vs. 11 seeds per 100 flowers) and three times more than geitonogamy (32 seeds per 100 flowers). Openly visited plants in the common garden set significantly fewer seeds per pod than plants from parent populations (P < 0.001). Wild plants were larger and likely older than the common garden transplants and probably received more frequent pollen deposition than did the common garden plants, which were manually pollinated only once. The pollinator guild was likely more depauperate in the common garden than in the wild populations (Watrous 2010; Watrous and Cane 2011).
Figure 6. Counts of seeds per pod compared across pollination treatments (from Watrous and Cane 2011). Autogamy: pollen was applied to the stigma of the same flower; geitonogamy: pollen was transferred between flowers on same plant; xenogamy: pollen was transferred between different plants grown from seed collected at the same location; distant xenogamy: pollen was transferred between different plants grown from seed collected from different ecoregions.
Pollinator visitation was evaluated for 16 forb species at primarily sagebrush sites in Oregon, California, Nevada, Idaho, and Utah (Cane and Love 2016). Researchers surveyed 11,399 basalt milkvetch plants at 61 sites and collected 1,097 bees, described as a “remarkably rich fauna of bees”. Almost 40% of the bees collected were mason bees (Osmia spp.), 33% were long-horned bees (Eucera spp.), 13.5% were bumble bees (Bombus spp.), each of theses bees were 2 to 3%: small mason bees (Hoplitis spp.), carder bees (Anthidium spp.), digger bees (Anthophora spp.), furrow bees (Halictus spp.), or sweat bees (Lasioglossum spp.), and each of these bees were 0.8%: striped sweat bees (Agapostemon spp.), mining bees (Andrena spp.), honey bees (Apis spp.), mason bees (Ashmeadiella spp.), digger bees (Habropoda spp.), leafcutter bees (Megachile spp.), mourning bees (Melecta spp.), cuckoo bees (Nomada spp.), or fairy bees (Perdita spp.) (Cane and Love 2016). The diversity of mason bees collected at basalt milkvetch represents a quarter of the species known to occur in North America (Watrous and Cane 2011).
In another survey of basalt milkvetch plants, findings were similar to the above with mason bees comprising 34 of the 77 species collected. Bees were sampled on wild and cultivated plants from 26 populations in Oregon, California, Nevada, Idaho, and Utah. Surveys occurred between 1000 and 1630 hours when bees were most active. The most widely collected bees were Bruner’s mason bee (Osmia bruneri), hypocrit summer mason bee (Hoplitis hypocrita), long-horned bee (Eucera frater), Hunt bumblebee (Bombus huntii), black-faced mason bee (O. nigrifrons) and central bumblebee (B. centralis). The bee species collected represent a range of nesting biologies including surface (2%), cavity (28%), ground (41%), and unknown (28%) nesting biologies (Watrous 2010).
Ecology
Basalt milkvetch has a moderate to long lifespan (Johnson et al. 2008; Eldredge et al. 2013; Shock et al. 2018b). Stems sprout in early spring and reach reproductive maturity by late spring or summer (May to July) (Johnson et al. 2008; Shock et al. 2018b; Ogle et al. 2019). Basalt milkvetch is considered a good seed producer and grows in high densities on recently burned sites (D.A. Johnson, unpublished data cited in [Bhattarai 2007]).
Seed And Seedling Ecology
Basalt milkvetch seed production is limited by seed predation (Youtie and Miller 1986; Cane et al. 2013). Seed feeding beetles (Acanthoscelides fraterculus, A. pullus, Apion amaurum, Tychius semisquamosus, and T. tectus) were found in seeds or flowers of plants growing throughout the species range (BC, WA, OR, CA, ID, NV, or UT). Acanthoscelides pullus was nearly ubiquitous in seed collections. The other beetles were found in fewer than 25% of collections (Cane et al. 2013).
Insect seed predation resulted in large seed crop reductions at three field sites in eastern Oregon (Youtie and Miller 1986, Table 1). Researchers monitored 25 plants at each western juniper/Wyoming big sagebrush/bluebunch wheatgrass-cheatgrass (Bromus tectorum) site. Plants were evaluated at least once a week from April through June in 1980 and 1981. Less than 7% of potential seed crops survived pre-dispersal insect predation in 1980 and almost 38% of seed survived in 1981. Pods were attacked by Torticidae larvae in 1980 and by tortrix moths (Torticidae) and true weevils (Cuculionidae) larvae in 1981. In 1980, predation was observed soon after May 15, and within 15 days, more than 80% of fruits showed signs of predation. In 1981, pod development and predation occurred over a longer time span. Although the number of damaged pods per plant increased in 1981, the total number of pods per plant increased and proportionately fewer pods were damaged (Youtie and Miller 1986). In 2005, similar seed production was found in wild populations sampled in Nevada and Idaho. Seed collected from four populations (100 pods/population) averaged 2.6 to 3.2 seeds/pod (Watrous and Cane 2011).
Table 1. Insect seed predation on basalt milkvetch plants in eastern Oregon (Youtie and Miller 1986).
Year | Plants/m2 | Flowers/plant | # pods/plant | # damaged pods/plant | # seeds/pod | Seeds produced/m2 | Seed survival/m2 |
1980 | 1.33 | 126 | 16* | 15* | 2.0 | 43* | 3* |
1981 | 1.33 | 152 | 40 | 26 | 3.8 | 202 | 76 |
* Significantly different between years.
Disturbance Ecology
Studies indicate that basalt milkvetch is disturbance tolerant, typically survives dormant season fires (Love and Cane 2019) and is an early colonizer of burned sites (Miller personal communication in [Jones et al. 2016]).
In fire studies, basalt milkvetch plants largely survived burning and were more abundant on burned than unburned sites when fires occurred in the dormant season. Three-year-old plants were fire tolerant when controlled burning was evaluated for field-grown plants at Oregon State University’s Malheur Experiment Station in Ontario, Oregon (Love and Cane 2019). Individual plants were burned with a propane burner in mid- to late-August when plants were well into senescence. Burning treatments were very low 120 ˚F (49 ˚C), low 135 ˚F (57 ˚C), moderate 151 ˚F (66 ˚C), high 248 ˚F (102 ˚C), and very high 264 ˚F (129 ˚C) temperatures at 0.8 inch (2 cm) below the soil surface. Of the 112 experimental plants, all 17 unburned plants survived through the year as did 81 of the 95 burned plants (85% survival). Mortality was less than 10% with very low, low, and medium fire temperatures, and 25% or less with high and very high fire temperatures. In the first postfire year, 40% of the high temperature-burned plants bloomed compared to 87% for unburned plants (P = 0.04). More than 60% of very high, medium, low, and very low temperature-burned plants flowered and more than 80% of unburned plants flowered in postfire year 1. Flower production was lower for burned plants but was similar across fire intensities (46-272 pods/plant, P = 0.16) (Love and Cane 2019).
Basalt milkvetch abundance was unaffected or increased following an August fire in mid- to late-seral Wyoming big sagebrush (Bates et al. 2011). The study area was in the Sheepshead Mountains about 68 miles (110 km) southeast of Burns, Oregon. The fire killed Wyoming big sagebrush, but basalt milkvetch was among the species having unchanged or increased cover from pre-burn levels in the first three post-fire years (Bates et al. 2011).
In a comparison of the effects of spring and fall prescribed fires in central Oregon, basalt milkvetch frequency was greater on fall burned and lower on spring burned than unburned plots although differences were not significant (Table 2, Sapsis 1990). Prescribed fires burned in basin big sagebrush/Idaho fescue-bluebunch wheatgrass communities at John Day Fossil Beds National Monument. Fires were set in late September in 1987 and late May in 1988 and vegetation sampling occurred in June or July. Total fuel consumption was twice as great with fall than with spring burning (Sapsis 1990).
On Steens Mountain in southeastern Oregon changes in abundance of basalt milkvetch were evaluated before and after cutting and burning 80-year-old western juniper trees (Bates et al. 1998; Bates and Svejcar 2009). Abundance was evaluated beneath tree canopies and in the interspaces before and after cutting and after burning felled trees (Bates et al. 1998). Basalt milkvetch plants were top-killed when trees landed on them but often recovered when these trees were burned. Basal cover of basalt milkvetch was not significantly different between interspace and duff zones before cutting (August 1991), but after cutting (1992-93), cover was significantly greater in interspaces than in duff or debris zones. Duff zones were the areas formerly beneath tree canopies, interspace zones were not influenced by formerly standing or felled trees, and debris zones were beneath felled trees (Bates et al. 1998). In a later study, where felled trees were burned in the first (1992) or second winter (1993) after cutting, basalt milkvetch cover was greater on cut and burned than cut and unburned sites through 2000. By 2001, though, there were no differences in basalt milkvetch cover on cut and unburned and cut and burned plots (Bates and Svejcar 2009).
Wildlife And Livestock Use
Seeds of basalt milkvetch and other milkvetches (Astragalus spp.) are eaten by pronghorn (Antilocapra americana), bighorn sheep (Ovis canadensis) western game birds (quail [Coturnix spp.] and wild turkeys [Meleagris gallopavo]), other ground-foraging birds, and small mammals (Martin et al. 1951). In a study evaluating the use of woody vegetation (Wyoming big sagebrush and western juniper) by Spanish goats (Capra hircus) to increase the production of herbaceous vegetation, basalt milkvetch was a preferred goat food and one of the most visited forb species. This study occurred on the USDA ARS Northern Great Basin Experimental Range southwest of Burns, Oregon (Fajemisin et al. 1996).
In a review of 68 articles noting the importance of forbs to greater sage-grouse (Centrocercus urophasianus), 10 articles indicated milkvetches provided food for the birds or occurred in their habitats (Pennington et al. 2016). Luna et al. (2018) reported that greater sage-grouse eat the flowers and leaves of basalt milkvetch and that the plants also support invertebrates eaten by the birds. At the Hart Mountain National Antelope Refuge in Lake County, Oregon, greater sage-grouse chicks (≤ 10 wks old) fed on basalt milkvetch, which comprised 20% of the frequency and 4.5% of the aggregate mass of the chick’s diets (Pyle 1993).
In the Cascadia region, which includes the state of Washington and the adjacent areas of British Columbia, Idaho, and Oregon, milkvetches are host plants for a variety of pollinators, including clouded sulphur (Colias phylodice), orange sulphur (C. eurytheme), western tailed-blue (Cupido amyntula), eastern tailed-blue (C. comyntus), Anna’s blue (Plebejus anna), and Melissa blue (P. melissa) butterflies. Melissa blue prefers milkvetch hosts early in the season. Queen Alexandra’s sulphur (C. alexandra) uses basalt milkvetch specifically as a larval host (James and Nunnallee 2011).
Nutritional Value
Nutritional content of basalt milkvetch was evaluated in southeastern Oregon (native site, Fajemisin et al. 1996) (Table 2) and Providence, Utah (common garden, Bhattarai et al. 2008) (Table 3). Crude protein was more abundant in actively growing than dormant plants (no statistical comparison) when plants were evaluated in July and August in the Northern Great Basin Experimental Range located southwest of Burns, Oregon (Table 2, Fajemisin et al. 1996).
Swainsonine, a toxic alkaloid poisonous to livestock and often associated with Astragalus species, does not occur in toxic levels in basalt milkvetch (Bhattarai et al. 2008; Ralphs et al. 2008).
Table 2. Nutritional content of basalt milkvetch at two time periods in the Northern Great Basin Experimental Range located southwest of Burns, Oregon. Statistical significance not evaluated (Fajemisin et al. 1996).
Month, growth phase | Dry matter | Crude protein | Neutral detergent fiber | Acid detergent fiber | Acid detergent lignin | In-vitro dry matter disappearance | Ash |
——————————%—————————– | |||||||
Active growth phase (July) | 33.4 | 17.7 | 52.5 | 42.4 | 8.5 | 54.8 | 4.8 |
Dormant phase (Aug) | 45.1 | 7.3 | 60.1 | 47.7 | 10.1 | 50.7 | 5.3 |
Forage quality was assessed for 67 accessions of basalt milkvetch grown together in a common garden south of Ogden, Utah. Researchers found that forage quality was inversely correlated with biomass yield. Crude protein concentration was negatively correlated with biomass yield (P < 0.01), and neutral detergent fiber and acid detergent fiber, which indicate low forage quality, were positively correlated with biomass yield (P < 0.0001) (Table 3, Bhattarai 2007).
Table 3. Nutritional content of 67 basalt milkvetch accessions grown together in Providence, Utah (Bhattarai et al. 2008).
Crude protein content | Neutral detergent fiber | Acid detergent fiber |
Mean (range) g/kg dry matter | ||
147 (118-202) | 420 (361-461) | 348 (308-380) |
Ethnobotany
Thompson Indians considered many milkvetch species to be good forage for horses and deer (Odocoileus spp.) (Turner et al. 1990).
Horticulture
Basalt milkvetch is drought resistant, attractive to pollinators, and produces showy flowers, making it potentially useful for xeriscaping or other low maintenance landscaping like campgrounds, rest areas, and visitor centers. The NBR-1 germplasm release (see Releases section) may be of interest to gardeners and homeowners in the Intermountain Region (Johnson et al. 2008).
Revegetation Use
Basalt milkvetch often occurs in large colonies, has a broad ecological and geographic range, provides excellent wildlife forage, and attracts pollinators, especially Osmia bee species (Cane 2011; Watrous and Cane 2011; Ogle et al. 2019). These traits and its ability to add nitrogen to restoration sites, make basalt milkvetch an attractive restoration species for degraded big sagebrush, mountain brush, and pinyon-juniper communities receiving average annual precipitation of 8 in (20 cm) or more (Plummer 1977; Ogle et al. 2019). Based on the revegetation of disturbed sites by the Utah Division of Wildlife, establishment of basalt milkvetch from seed was rated as moderate, and establishment from transplants, natural spread by seed, growth rate, and soil stability were rated good (Plummer 1977). Once established, basalt milkvetch sprouts readily after fire. In some cases, plants were observed to produce new shoots from lateral roots, which may indicate resistance to and persistence with grazing pressure (Tilley et al. 2017).
Developing A Seed Supply
For restoration to be successful, the right seed needs to be planted in the right place at the right time. Coordinated planning and cooperation is required among all partners to select appropriate species and seed sources; these are determined by current or projected revegetation requirements and goals (PCA 2015).
Developing a seed supply begins with seed collection from native stands. Collection sites are determined by current or projected revegetation requirements and goals as well as through consultation with land managers and stakeholders. Collected seed is distributed for restoration or first increased in seed fields to provide a larger supply of seed (PCA 2015). Production of nursery stock requires less seed than large-scale seeding operations. Regardless of the size and complexity of any revegetation effort, seed certification is essential for tracking seed origin from collection through use (UCIA 2015).
Seed Sourcing
Because empirical seed zones are not currently available for basalt milkvetch, generalized provisional seed zones developed by Bower et al. (2014) may be used to select and deploy seed sources. These provisional seed zones identify areas of climatic similarity with comparable winter minimum temperature and aridity (annual heat to moisture index). In Figure 7, Omernik Level III Ecoregions (Omernik 1987) overlay the provisional seed zones to identify climatically similar but ecologically different areas. For site-specific disturbance regimes and restoration objectives, seed collection locations within a seed zone and ecoregion may be further limited by elevation, soil type, or other factors.
The Western Wildland Environmental Threat Assessment Center’s (USDA FS WWETAC 2017) Threat and Resource Mapping (TRM) Seed Zone application provides links to interactive mapping features useful for seed collection and deployment planning. The Climate Smart Restoration Tool (Richardson et al. 2020) can also guide revegetation planning, seed collection, and seed deployment, particularly when addressing climate change considerations.
Although broadly adapted and widely distributed as a species, genetic and phenotypic differences exist among basalt milkvetch populations. Based on 67 wildland site seed collections (mean 9.2 plants/collection) made in British Columbia, Washington, Oregon, California, Nevada, and Idaho, within-population genetic diversity was greatest for collections in Oregon and lowest for collections in British Columbia and central Nevada (Bushman et al. 2010). All 67 accessions were grown in two common gardens in Utah, one a silty clay loam site in Providence, and the other a gravelly loam site in Millville (Table 4, Bhattarai et al. 2008). Seedlings were 90 days old when transplanted to the common gardens in spring 2004 (see Nursery Production section for details). Accessions collected in close proximity were more similar morphologically than those collected from more distant locations. Plants from seed collected in north-central Oregon produced high biomass compared to plants from seed collected in Nevada, Washington, Utah, and parts of Idaho. Seed mass was positively correlated with biomass in 2005 (P < 0.0001) and 2006 (P < 0.0001), and plants from seed collected at higher elevations tended to produce heavier seeds and plant biomass. Number of stems was closely related to biomass yield, which was highly correlated with seed yield (P < 0.0001). Seed yield ranged from 0 to 9.5 g/plot in 2005, and 0 to 7.5 g/plot in 2006. Generally, accessions with high biomass in June had high biomass in October following regrowth after clipping to 2 in (5 cm) at about 50% bloom. Winter mortality varied in both gardens. At Providence, winter mortality ranged from 0 to 30% and averaged 9% in 2005 and ranged from 0 to 73% and averaged 23% in 2006. At Millville, winter mortality ranged from 7% to 53% and averaged 25% in 2005 and ranged from 13% to 83% and averaged 35% in 2006. (Bhattarai 2007; Bhattarai et al. 2008).
Table 4. Average and range values* for plant characteristics of 67 basalt milkvetch accessions grown in two common gardens in Utah (Bhattarai et al. 2008).
Site | Year | Regrowth biomass (g/plot in Oct) | Plant height (in) | Stems (no) | Inflorescences (no) | Vigor score (scale: 0-9) |
Providence | 2005 | 7 (1-69) | 10 (6-15) | 4 (2-10) | 4 (0-17) | 5 (3-7) |
2006 | 14 (1-59) | 10 (6-13) | 14 (4-32) | 19 (1-82) | 4 (2-5) | |
Millville | 2005 | 3 (0-17) | 10 (7-15) | 5 (2-13) | 4 (0-30) | 5 (3-7) |
2006 | ND | 11 (7-14) | 21 (6-37) | 41 (2-79) | 4 (2-7) |
*Within each common garden, plant biomass, plant height, number of stems, number of inflorescences, and vigor scores varied significantly (P < 0.01) among the accessions in both years.
ND – No data. Plants had only very minimal regrowth from August to October in 2006 at Millville site.
To identify regional seed sources for basalt milkvetch Bushman et al. (2010) characterized within-collection diversity, identified genetically differentiated groups, and tested isolation distance for the 67 widely distributed basalt milkvetch collections described in detail above. Within-population diversity was greatest for collections in Oregon and lowest for collections in British Columbia and central Nevada. Five genetically differentiated groups were identified: one strong group in central Nevada, one strong group in British Columbia, and three weaker groups for all other collections in Oregon, Washington, California, Idaho, and Nevada. Findings also suggested a trend of increasing diversity in central Oregon. Study results revealed that geographic distance contributed to genetic differentiation, with structured populations occurring in the northernmost and southernmost groups of collections of basalt milkvetch (Bushman et al. 2010).
Occurrence Map
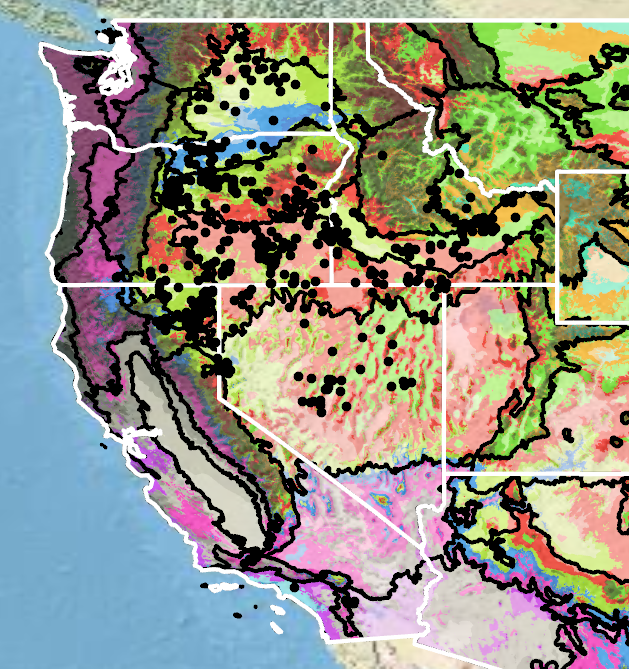
Figure 7. Distribution of basalt milkvetch (black circles) based on geo-referenced herbarium specimens and observational data from 1875–2023 (CPNWH 2020; SEINet 2020; USDI USGS 2020). Generalized provisional seed zones (colored regions) (Bower et al. 2014) are overlain by Omernik Level III Ecoregions (black outlines) (Omernik 1987; USDI EPA 2018). Interactive maps, legends, and a mobile app are available (USDA FS WWETAC 2017; www.fs.fed.us/wwetac/threat-map/TRMSeedZoneMapper2.php?). Map prepared by S. Barga and B. Bautch-Breitung, USDA Forest Service, RMRS.
Releases
A basalt milkvetch germplasm (NBR-1) was released in 2008 by USDA-ARS Forage and Range Research Lab in cooperation with Utah Agricultural Experiment Station and Utah State University (Johnson et al. 2008; Tilley et al. 2017). Twelve of the 67 accessions in the common garden described earlier (see Seed Sourcing section) were used to develop the germplasm (Johnson et al. 2008). Seed from the 12 accessions was bulked in equal weights to form the G0 generation of NBR-1 Germplasm (Johnson et al. 2008). NBR-1 Germplasm accessions came from seed collected from sites in the Northern Basin and Range Level III ecoregion. Collection sites were currently or previously dominated by big sagebrush or juniper, ranged from 4,049 to 6,148 ft (1,234-1,874 m) in elevation, and occupied basalt-derived sandy, loamy, or gravelly soils. Because not all Northern Basin and Range seed accessions were included in germplasm development, NBR-1 is a manipulated-track plant material selection. Material selection was based on high seed yields and low nitrotoxin and selenium content (Johnson et al. 2008). Based on assessments of genetic variation and phenotypic expression from common garden studies and AFLP evaluation, NBR-1 Germplasm represents the largest meta-population of the 67 accessions evaluated (Bushman et al. 2010). NBR-1 Germplasm was developed for use in post-fire reclamation, native plant community restoration, wildlife habitat improvement, and plant diversity enhancement in the Northern Basin and Range Ecoregion (nw UT, n NV, s ID, se OR, and ne CA) where annual precipitation averages 8 to 18 in (200-460 mm) (Johnson et al. 2008; Tilley et al. 2017).
Wildland Seed Collection
Basalt milkvetch produces flowers over a long period (Shock et al. 2018b), which suggests uneven seed ripening. Although Barneby (1989) notes that pods are often shed unopened and pod dehiscence is delayed, high seed pod shatter made seed recovery difficult and reduced seed yield in agricultural studies (Fig. 8) (Shock et al. 2018a). Seed predation is a common occurrence, so evaluating a collection site prior to investing time in harvesting is recommended. Because of the high levels of seed predation, collections will likely need to be large and may contain considerable trash that must be removed during cleaning.
Figure 8. Basalt milkvetch pods collected from plants in Oregon. Photo: USDI BLM OR010, SOS.
Wildland Seed Certification
Verification of species and tracking of geographic source is necessary whether wildland seed is collected for immediate project use or as stock seed for cultivated increase. This official Source Identification process can be accomplished by following procedures established by the Association of Official Seed Certifying Agencies (AOSCA) Pre-Variety Germplasm Program (UCIA 2015; Young et al. 2020). Wildland seed collectors should become acquainted with state certification agency procedures, regulations, and deadlines in the states where they collect.
If wildland-collected seed is to be sold for direct use in ecological restoration projects, collectors must apply for Source-Identified certification prior to making collections. Pre-collection applications, site inspections, and species and seed amount verification are handled by the AOSCA member state agency where seed collections will be made (see listings at AOSCA.org).
If wildland seed collected by a grower or private collector is to be used as stock seed for planting cultivated seed fields or for nursery propagation (See Agricultural Seed Field Certification section), detailed information regarding the collection site and collecting procedures must be provided when applying for certification. Photos and herbarium specimens may be required. Germplasm accessions acquired within established protocols of recognized public agencies, however, are normally eligible to enter the certification process as stock seed without routine certification agency site inspections. For contract grow-outs, however, this collection site information must be provided to the grower to enable certification.
Collection Timing
Based on seed harvests made by the USDI BLM Seeds of Success collectors, seed has been harvested from June 30 to August 15 (Fig. 9) (USDI BLM SOS 2017). Harvest data was available for 17 collections made in 6 years from 2002 to 2016 in California (1), Idaho (3), and Oregon (13). At three sites, a second collection was made about a week after the first. The earliest collection occurred on June, 30, 2015, in Malheur County, Oregon, at 4,641 ft (1,415 m) in elevation. The latest collection was made on August 15, 2011, in Lake County, Oregon at 5,199 ft (1,585 m) in elevation. In the single year (2011) with the most collections made (6), the earliest was on July 11 in Malheur County, Oregon at 3,000 ft (914 m) in elevation and the latest was August 15 in Lake County, Oregon at 5,199 ft (1,585 m) in elevation, suggesting that phenology and seed maturation were later at upper elevations (USDI BLM SOS 2017).
Figure 9. Basalt milkvetch pod and seeds (scale in inches). Photo: USDI BLM OR010, SOS.
Collection Methods
Pods are collected by hand stripping them from the inflorescence or by clipping the entire inflorescence. Collections sites should be scouted to inform collection date and to evaluate the level of seed predation. Prior to harvesting seed, open the pods from several plants to determine predation.
Several collection guidelines and methods should be followed to maximize the genetic diversity of wildland collections: 1) collect seed from a minimum of 50 randomly selected plants; 2) collect from widely separated individuals throughout a population without favoring the most robust or avoiding small stature plants; and 3) collect from all microsites including habitat edges (Basey et al. 2015). General collecting recommendations and guidelines are provided in online manuals (e.g., ENSCONET 2009; USDI BLM SOS 2021).
It is critical that wildland seed collection does not impact the sustainability of native plant populations. Collectors should take no more than 20% of the viable seed available at the time of harvest (USDI BLM SOS 2021). Additionally, care must be taken to avoid the inadvertent collection of weedy species, particularly those that produce seeds similar in shape and size to those of basalt milkvetch.
Post-Collection Management
Wildland seed collections should be kept in a cool, dry location free from rodents. Bhattarai (2007) placed insecticidal strips into each bag of collected seed to minimize seed damage from associated insects. Placing collections in a freezer is also used to control insects.
Seed Cleaning
Bhattarai (2007) used the following procedure to clean basalt milkvetch seed collected thoughout the range of the species (Fig. 10). Seed was dried for 2 months before being processed through a Winterseiger Seed Thresher (Model LD180, Des Moines, IA) using size 9K and A screens (Seedburo Equipment Co., Chicago, IL). Seed cleaning was finished by processing through a seed blower (Bhattaria 2007). Tracking historic bushel weights can be useful for estimating seed quality in the cleaning process (Tilley and Ogle 2011). Seed lots not meeting expected bushel weights (60.4 lbs/bushel [777.460 kg/m3 or .777460 kg/l] for basalt milkvetch) can be further processed using higher airflow rates to remove lighter unfilled seed. Multiplying the pounds per bushel value by 12.9 provides a gram per liter estimate (Tilley and Ogle 2011).
Figure 10. Basalt milkvetch seed under magnification. Photo: USDA USFS, Bend Seed Extractory.
Seed Storage
Seed is likely orthodox because a high proportion of mature seed is hard and likely long-lived, but best storage practices and seed longevity with storage were not provided in the reviewed literature. Bhattarai (2007) stored seed in the dark at 37 °F (3 °C) and 20 to 25% relative humidity. In the germination studies below, seed viability and germination potential were maintained after room temperature and dry, cool storage for about a year (Jones et al. 2016; Kildisheva et al. 2018).
Seed Testing
There are no AOSA guidelines or methods provided for testing the viability or germinability of milkvetch seed (AOSA 2010, 2016).
Germination Biology
Basalt milkvetch seed exhibits combinational physical and physiological dormancy (Jones et al. 2016; Kildisheva et al. 2019). Germination requires scarification of the seed. Studies have tested various methods for breaking seed dormancy, and pneumatic mechanical scarification was best.
Ransom (2014) obtained small increases in basalt milkvetch germination by scarifying seed in concentrated sulfuric acid for 30 minutes. Increasing the exposure to sulfuric acid to 45 minutes resulted in injury to emerging shoots. Germination was increased to 80% when 30 minutes of acid scarification was followed by 10 to 20 days of cold exposure (39-43 °F [4-6 °C]). Jones et al. (2016) tested a combination of scarification (sandpaper), germination substrate (moist sand or blotter paper), and stratification (3 wks in dark at 41 °F [5 °C]) treatments on seed collected from fields growing near Millville, Utah, and stored at room temperature for up to 12 months. Treated and untreated seeds were germinated at 72 °F (22 °C) with ambient daylight conditions. Germination percentages were low overall. Five percent or less of seed germinated without stratification or scarification, 20% or less with stratification or scarification alone, and 30% or less with prechilling and scarification. Seed viability averaged 94.5%, and scarification did not impact viability. For all treatment combinations, the number of seeds germinating increased over time and did not plateau by 10 weeks. Sandpaper treatments left about 75% of seed hard, suggesting more aggressive scarification was needed (Jones et al. 2016).
In a comprehensive germination study, Kildisheva et al. (2019) evaluated 22 treatments including exposure to freezing and near boiling temperatures and various types and durations of abrasion, but only abrasion resulted in large increases in basalt milkvetch germination. Seed permeability, imbition, and germination were evaluated after treatments of wet heat (5-300 s in 194 °F [90 °C] water), freezing + wet heat (2 hr at -112 or -4 °F [-80 or -20 °C] then 5 or 30 s in 194 °F [90 °C] water), freeze-thaw cycles (2 hr at -112 or -4 °F [-80 or -20 °C] then 2 hr at 73 °F [23 °C] repeated 1-6 times), and six abrasion-based treatments: pneumatic scarification (10-160 s in a Mater seed scarifier, Corvallis, OR) and manual scarification (120-grit sand paper). Basalt milkvetch seed required at least 40 seconds of pneumatic scarification to significantly improve germination (P = 0.0471) over controls. Scarification for 160 seconds resulted in nearly complete germination (95%). Seed used in this study was collected in northeastern Utah in July 2015 and stored in dry, cool conditions until treatments were applied in summer 2016 (Kildisheva et al. 2018).
Wildland Seed Yield And Quality
Post-cleaning seed yield and quality of seed lots collected in the Intermountain region are provided in Table 5 (USDA FS BSE 2017). The results indicate that basalt milkvetch seed can generally be cleaned to high levels of purity and seed fill, but the fill and viability of fresh seed is variable. Seeds/lb reported for basalt milkvetch found in other sources (113,854-120,000 seeds/lb) fell within the range reported in Table 5 (Ogle et al. 2011; SER, INSR, and RBGK 2023).
Table 5. Seed yield and quality of basalt milkvetch seed lots collected in the Intermountain region, cleaned by the Bend Seed Extractory, and tested by the Oregon State Seed Laboratory or the USDA Forest Service National Seed Laboratory (USDA FS BSE 2017).
Seed lot characteristic | Mean | Range | Samples (no.) |
Bulk weight (lbs) | 2.9 | 0.11–29 | 23 |
Clean weight (lbs) | 0.30 | 0.008–2.6 | 23 |
Clean-out ratio | 0.13 | 0.02–0.33 | 23 |
Purity (%) | 96 | 82–99 | 23 |
Fill (%)¹ | 82 | 38–99 | 23 |
Viability (%)² | 90 | 70–97 | 21 |
Seeds/lb | 101,845 | 71,000–132,476 | 23 |
Pure live seeds/lb | 88,758 | 61,152–115,413 | 21 |
¹ 100 seed X-ray test
² Tetrazolium chloride test
Marketing Standards
Acceptable seed purity, viability, and germination specifications vary with revegetation plans. Purity needs are highest for precision seeding equipment used in nurseries, while some rangeland seeding equipment handles less clean seed quite well.
Agricultural Seed Production
Several studies and research organizations have investigated important techniques for successfully producing basalt milkvetch seed using agricultural methods. These have evaluated various seed pretreatments, seeding methods, seeding depths, and irrigation treatments. Although harvestable seed was produced, these studies revealed that a number of challenges to agricultural seed production remain. However, Youtie and Miller (1986) found that heavy insect predation in native stands suggests that dependence on wild seed sources for revegetation efforts is probably not reasonable.
Agricultural Seed Certification
In order to minimize genetic changes in specific accessions of native species when increased in cultivated fields, it is essential to track the geographic source and prevent inadvertent hybridization or selection pressure. This is accomplished by following third party seed certification protocols for Pre-Variety Germplasm (PVG) as established by the Association of Official Seed Certification Agencies (AOSCA). AOSCA members in the U.S., Canada, and other countries administer PVG requirements and standards that track the source and generation of planting stock. Field and cleaning facility inspections then monitor stand establishment, proper isolation distances, control of prohibited weeds, seed harvesting, cleaning, sampling, testing, and labeling for commercial sales (UCIA 2015; Young et al. 2020).
Seed growers apply for certification of their production fields prior to planting and plant only certified stock seed of an allowed generation (usually less than four). The systematic and sequential tracking through the certification process requires preplanning, knowing state regulations and deadlines, and is most smoothly navigated by working closely with state certification agency personnel. See the Wildland Seed Certification section for more information on stock seed sourcing.
Site Preparation
Firm weed-free beds are used to establish seed production fields. Greenhouse studies identified a commercially available rhizobia strain with exceptional infectiveness and effectiveness for nitrogen fixation of basalt milkvetch (Johnson et al. 2008).
Seed Pretreatments
Because basalt milkvetch seed exhibits both physical and physiological dormancy, germination can be erratic and poor, which is ill suited to agricultural seed production. Field studies tested various scarification and stratification methods and season of seeding to maximize emergence, and establishment but results were mixed (Jones et al. 2016; Bushman et al. 2019).
In 3 years of seeding at three sites in north-central and western Oregon, Bushman et al. (2019) found that emergence of basalt milkvetch was greatest for scarified seed that was planted in fall. The study monitored emergence following planting of both basalt milkvetch and Blue Mountain prairie clover (Dalea ornata). Total seedling emergence was low but greatest in the spring with highest precipitation levels (> 3 in [75 mm] March-May). Scarification treatments exposed seed to 5N sulfuric acid for 5 minutes. Seeds were planted with a Wintersteiger (Williston, Vermont) singlerow seeder at a 0.5 in (1.3 cm) depth. The seedbed at the Ontario location was tilled prior to each planting, the seedbed at the Powell Butte location was tilled only prior to the first fall planting, and the Clarno site had no seedbed preparation. Total seedling emergence was greatest at the Powell Butte site (>6 seedlings/row of 240 seeds) (Bushman et al. 2019).
A variety of scarification and combined scarification and stratification treatments were tested to increase emergence at two Utah field locations. Seed was collected from Millville Farm, Utah, in summer 2010 and stored at 39 °F (4 °C) until treatments in early April 2014. Seed treatments were: 1) untreated control, 2) mechanical scarification (6 s in a Forsberg Line debearder [Fred Forsberg & Sons, Inc., Thief River Falls, MN] having four internal paddles and medium-grit emery paper), 3) boiling scarification (10s in boiling water), 4) acid scarification (5 m in 97% sulfuric acid), and 5) acid scarification + stratification (14 d in moist sand in the dark at 39 °F [4 °C]). Emergence of acid and boil treated seed was not different from controls at either site. Emergence of seed treated with acid + prechill or sandpaper scarification was significantly greater than control seed (P < 0.01) at both sites. At both locations, density of the acid, boil, and control seedlings remained stable across the evaluation period (July and September), but there was attrition for acid + prechill and sandpaper seedlings. So, although emergence was improved by the acid + prechill or sandpaper scarification, not all emerged seedlings established. In September or October, there were still more than double the density of acid + prechill and sandpaper seedlings than control, acid, or boil seedlings at either site (Jones et al. 2016).
Weed Management
At OSU MES, cultivation or hand rouging was used to control weeds in seed production plots (Shock et al. 2018), but in two years herbicide treatments were also used. Plots were broadcast treated on October 27, 2016, with pendimethalin (1 lb ai/ac) and on April 21, 2017, with sethoxydim (30 oz/ac) and pendimethalin (1 lb ai/ac) (Shock et al. 2018a).
Ransom (2014) tested the effect of preemergent herbicides on germination of basalt milkvetch. While several herbicide treatments (imazapic, pendimethalin, and dimethenamid) did not affect germination, they often resulted in fewer leaves per pot than controls. In this greenhouse study, 4-in (10-cm) pots were filled with native silt loam Millville soil (Utah State University Greenville Research Farm). Twelve basalt milkvetch seeds were scarified with concentrated sulfuric acid for 30 minutes and added to each pot. Pots were sprayed with herbicide then put in the refrigerator 39 °F (4 °C). After 11 days of cold, pots were moved to a greenhouse with shade cloth (77 °F [25 °C]) day and 73 °F [23 °C]) night (Ransom 2014).
Many preemergent herbicides were field tested at OSU MES (Shock et al. 2011, Table 6). Emergence was reduced by about 50% or more with herbicide treatments, but treatments were not statistically different, and stand emergence was uneven regardless of the treatment. Herbicides were applied on November 30, 2009, and seeding occurred on December 1, 2009. Seed was surface seeded then covered with thin layer of sawdust. Emergence was evaluated in spring 2010 (Shock et al. 2011).
Table 6. Preemergent herbicide effects on the emergence of basalt milkvetch at Oregon State University Malheur Experiment Station in Ontario, Oregon (Shock et al. 2011).
Treatment | Rate (lb ai/ac) | % Emergence* |
Untreated | None | 66 |
Bensulide | 5 | 32 |
Pronamide | 1 | 20 |
Trifluralin | 0.375 | 34 |
Pendimethalin | 0.75 | 30 |
Benefin | 1.2 | 33 |
Dimethenamid | 0.656 | 31 |
Linuron | 0.5 | 27 |
*No statistically significant differences in emergence by treatment.
At seed production plots at Utah State University Greenville Research Farm, injury of established basalt milkvetch plants was evaluated after a variety of herbicide treatments. Although several treatments resulted in little to no injury or change in growth (pendimethalin, dimethenamid, flumioxazin, metribuzin, octanoic acid ester of bromoxynil), the impacts on seed production were not evaluated. Plots were established by planting nursery stock in 2005. Herbicide treatments were applied on May 13, 2008. Injury was evaluated on May 23 and again on July 25 (Ransom and Edvarchuk 2009).
Seeding
Establishment of basalt milkvetch from seed can be poor or variable. Transplanting is often preferred over seeding for establishment of high density stands (Tilley et al. 2017). Transplanting success, however, can also be variable with high rates of mortality possible (Jones et al. 2016).
Effects of timing of seeding (fall vs. spring), seed treatments (fungicide vs. no treatment), scarification (acid scarification vs. no treatment), and protection (row cover vs. no cover) were evaluated at OSU MES (Shock et al. 2013). Stand emergence was highest (36.2%) for fall seeded scarified seed with row cover and no fungicide seed pretreatment. Seed was planted into prepared fields on November 11, 2011, or February 28, 2012. Emergence was poor overall but was greater for seeds planted in the fall than in the spring. Emergence of scarified seed (5 m in 98% sulfuric acid) was greater than nonscarified seed. Emergence of fungicide-treated seeds (metalaxyl [100 g] and captan [100 g] mixed with 1 L of water) was lower than untreated seeds. Row cover (N-sulate, De Witt Co, Sikeston, MO) that protected seeded areas until early April also improved emergence (Shock et al. 2013).
At OSU MES, the following protocol was used to seed production plots that were used to test irrigation effects on seed production. Pooled accessions of wildland seed were deposited on the soil surface using a custom small-plot grain drill with disk openers (213-328 seeds/ft [65-100 seeds/m] of row) in rows that were 30 in (76 cm) apart. Seed was subsequently covered with sawdust at rate of 7 g/foot (24 g/m) of row and then by row cover (N-sulate, DeWitt Co, Inc. Sikeston, MO), which was removed in April. Soil at OSU MES is Nyssa silt loam with a pH of 8.3 and 1.1% organic matter. Seeding occurred in late November 2009 (Shock et al. 2018b, 2018a).
Planting
Survival was variable but mostly good (>70%) when greenhouse-grown basalt milkvetch plants were used to establish stands at two common garden sites in Utah (North Logan and Kaysville) (Table 7, Kratsch et al. 2010). Plants were outplanted in spring 2008, watered as needed in the first growing season but not after that. Plants of both accessions were in nearly full bloom in early June 2009 (Kratsch et al. 2010).
Table 7. First year survival of basalt milkvetch seedlings outplanted in spring 2008 to two sites in Utah. Seed came from four pooled wild accessions collected in north-central Oregon (nc OR) or was obtained from L & H Seed Company (LH) (Kratsch et al. 2010).
Location | Accession | Winter 2009 survival* | Summer 2009 survival |
North Logan, UT | nc OR | 83% | 80% |
LH | 28% | 60% | |
Kaysville, UT | nc OR | 83% | No data |
LH | 78% | No data |
*Statistical significance for survival at the two sites was not evaluated.
Irrigation
Basalt milkvetch seed production did not respond to irrigation treatments in seed production plots at OSU MES where seed yields were low because of low density stand establishment, seed beetle predation, and reliance on a single seed harvest (Shock et al. 2018b, 2018a). Seed yield and plant water relations were evaluated for 5 years (2011-2015). The OSU MES site occurs at 2,260 ft (689 m) elevation, soils are silt loams, and annual precipitation averages 9.8 in (249 mm) (range: 7-11.5 in [179-292 mm]). Irrigation studies added 0, 4, and 8 inches (0, 100, 200 mm) of water through a subsurface drip irrigation system (12 in [30 cm] deep), with four bi-weekly increments starting at the time of flower bud formation (Table 8) (Shock et al. 2018b, 2018a). Plots were seeded in 2009 and irrigated for 24 hours on December 2, 2009, because of very dry conditions. Basalt milkvetch seed yield did not respond to irrigation from 2011 to 2015 (Table 9). January to June precipitation at the study area was close to average in 2012, 2014, and 2015, higher than average in 2011, and lower than average in 2013 (Table 9) (Shock et al. 2018b, 2018a).
Table 8. Flowering timing as related to timing of irrigation of basalt milkvetch seed production plots growing at Oregon State University’s Malheur Experiment Station in Ontario, OR (Shock et al. 2018b)
Year | Flowering | Irrigation | ||||
Start | Peak | End | Start | End | Harvest | |
2011 | May 20 | May 26 | June 30 | May 13 | June 23 | July 18 |
2012 | Apr 28 | May 23 | June 19 | May 11 | June 21 | July 5 |
2013 | May 3 | May 10 | May 25 | May 8 | June 19 | June 28 |
2014 | May 5 | May 13 | May 28 | Apr 29 | June 10 | June 24 |
2015 | Apr 17 | May 13 | June 1 | Apr 21 | June 3 | June 16 |
Table 9. Seed yield of basalt milkvetch with and without irrigation of seed production plots growing at Oregon State University’s Malheur Experiment Station in Ontario, OR (Shock et al. 2018b, 2018a).
Year | Supplemental irrigation (in/season*) | Fall + Winter + Spring (in) | Growing degree days (10-30 °C) Jan-June | ||
0 | 4 | 8 | |||
———Seed yield (lbs/ac)**——— | |||||
2011 | 86.9 | 98.3 | 74.0 | 14.4 | 476 |
2012* | 22.7 | 12.6 | 16.1 | 8.4 | 682 |
2013 | 8.5 | 9.8 | 6.1 | 5.3 | 733 |
2014 | 56.6 | 79.2 | 71.8 | 8.1 | 741 |
2015 | 17.8 | 12.5 | 11.6 | 10.4 | 895 |
Average | 38.6 | 35.2 | 36.0 | NA | NA |
*On April 23, 2012, 5 lb Fe/ac (5.6 kg/ha) was applied as liquid fertilizer through irrigation drip tape (Shock et al. 2014).
**Seed yield was not statistically significant between irrigation treatments in any year.
Pollinator Management
Cross pollination by bees is necessary for good seed production (Watrous and Cane 2011). In surveys of wild and cultivated basalt milkvetch populations in Oregon, California, Idaho, Nevada, and Utah, mason bees comprised 34 of the 77 bee species collected. Bees represented a range of nesting biologies (Watrous 2010). Two percent were surface, 28% were cavity, 41% were ground nesting, and the rest had unknown nesting requirements. Based on the evaluation of flower handling, Bruner’s mason bees and hypocrit summer mason bees were identified as prospective pollinators for management in association with rehabilitation efforts with basalt milkvetch. These bees are cavity nesters, occur throughout the range of basalt milkvetch, and had high frequencies of stigmatic contact. Basic nesting biology of Bruner’s mason bee is known, and management of the species is being researched at the USDA, Agricultural Research Service Pollinating Insect-Biology, Management, Systematics Research Unit in Logan, Utah. Manageable honey bees (Apis mellifera) (Cane et al. 2012), were the poorest pollinators surveyed and frequently failed to contact the pistil of basalt milkvetch flowers (Watrous 2010). While research continues on procedures for managing, moving, and sustaining pollinator populations in forb seed cultivation, encouraging and sustaining native bee populations where present can benefit production of many native plant crops (Cane 2008).
Pest Management
Seed feeding beetles are common in wild stands (Youtie and Miller 1986; Cane et al. 2013) and can cause heavy seed loses in cultivated stands. Acanthoscelides fraterculus, A. pullus, Apion amaurum, Tychius semisquamosus, and T. tectus were found in flowers or collected seed of plants growing throughout the range of the species. A. pullus was nearly ubiquitous in seed collections, while the others occurred in fewer than 25% of seed collections (Cane et al. 2013). In seed production plots at OSU MES, damage from seed weevils was extensive in 2013 and 2014. Pesticide treatments (bifenthrin (0.1 lb ai/ac [6.4 oz/ac]) and novaluron (0.08 lb ai/ac [12 oz/ac]) were used to treat seed beetles in plots in May 2015. Pesticides were applied in the evening to minimize harm to pollinators and were successful in limiting seed beetles during the 2016 and 2017 flowering period (Shock et al. 2018a). Powdery mildew (Leveillula papilionacearum) was also identified on basalt milkvetch at OSU MES, but damage or impact was not reported (Mohan and Shock 2014).
Seed Harvesting
The upright stems produced by basalt milkvetch make seed harvest easier than for other low growing milkvetch species. Yet, seed shatter is an obstacle to high volume seed production. Pod-sealing agents and growing plants on fabric are suggested for improving the yields of cultivated seed (Tilley et al. 2017). At OSU MES, basalt milkvetch flowers matured over a long period and seed yields were low. Seed was harvested with a single once over mechanical treatment (Shock et al. 2018b).
Seed Yields And Stand Life
Basalt milkvetch produced harvestable seed in year 2 and stands produced seed for all 4 years of evaluation in research plots at OSU MES. Low seed yields were the result of poor stand establishment and high seed pod shatter (Shock et al. 2018a).
Nursery Practice
Based on experience, Bushman and colleagues (Bushman et al. 2015; Bushman 2023, personal communication) referred to basalt milkvetch as “a straggler filipes” because of its notoriously slow germination and establishment. Best germination and establishment was achieved by keeping seed in cool wet sand for about 3 weeks.
Bhattarai (2007) grew 67 accessions of basalt milkvetch from seed. Collected seed was stored in dark conditions at 37 °F (3 °C) with 20 to 25% relative humidity until it was germinated on moist blotter paper. Germinants were placed in cone-tainers (1.5 in [4 cm] diameter, 8.7 in [22 cm] long) filled with a 3:1 sand:peat mixture. Cone-tainers were kept in a greenhouse (86 °F [30 °C] day, 59 °F [15 °C] night). After 1 week of growth, homogenized sieved field soil (5 mm) was added to each cone-tainer for rhizobial inoculation. After 3 weeks, a slurry of common rhizobial inoculum (Astragalus Special No. 1, Nitragin Co., Milwaukee, WI) was also added to each cone-tainer. After about 90 days of greenhouse growth, cone-tainers were transplanted into a common garden. In additional tests, researchers isolated six rhizobial strains from the root nodules of basalt milkvetch and identified one strain that exhibited exceptional infectiveness and effectiveness for nitrogen fixation. An inoculant of this strain is commercially available (Johnson et al. 2008).
Wildland Seeding And Planting
The recommendations for seeding basalt milkvetch in wildland settings include a full seeding rate of 9 lbs/ac (10 kg/ha) (Ogle et al. 2019) and a seeding depth of 0.25 to 0.5 in (0.6-1.3 cm) in medium to coarse or silty sandy soils where annual precipitation averages at least 8 in (200 mm) (Ogle et al. 2011, 2012). Basalt milkvetch should be a minor component (10% or less) of a restoration seed mix (Ogle et al. 2012). Post-seeding management should be based on the key species in the establishing community, and grazing should be deferred for at least 2 years (Ogle et al. 2012; Tilley et al. 2017).
A greenhouse experiment suggests that basalt milkvetch may be a good species to use following herbicide treatments to control weed competition (de Graaff 2016). Soil was collected up to 4 in (10 cm) deep from burned and unburned degraded big sagebrush ecosystems at Morley Nelson Birds of Prey in southwestern Idaho. Scarified basalt milkvetch seed (3 min with 220 grit sandpaper) was seeded 3.5 mm deep into field soils that were treated with 140, 280, and 560 g ai/ha of imazapic. Plants were grown for 41 days at 59 to 77 °F (15-25 °C). Standing biomass of basalt milkvetch treated with imazapic ranged from about 0.3 to 0.5 g in burned or unburned soils and was close to 0.7 g for untreated plants in burned or unburned soils, which often represented a statistically significant reduction (P < 0.05). These negative impacts, however, were much less than what was observed for the other species evaluated (common yarrow [Achillea millefolium] and scarlet globemallow [Sphaeralcea coccinea]). In another experiment that evaluated the timing of imazapic applications, there was no significant impact to basalt milkvetch standing biomass with 280 g ai/ha applications made 2, 4, 8, and 16 weeks before seeding the treated soils (de Graaff 2016).
Several studies compared emergence and establishment of basalt milkvetch with various seed treatments, seeding methods, and seeding depths. In many cases, establishment was poor, and site often played a role in the best seeding methods or treatments. Basalt milkvetch was seeded as part of a mix at Saylor Creek in Elmore County, Idaho. The Wyoming big sagebrush site burned in June 2010 and was seeded in October 2010. Soils were loamy (11% sand, 54% silt, 35% clay). The seeding rate for basalt milkvetch was 1.3 PLS/linear ft (4.3 PLS/linear m). Seeding was compared using a conventional rangeland drill with irregular seeding depths and a minimum-till drill with a 1 in (2.5 cm) seeding depth. Density of basalt milkvetch was not different by seeding method in year 1 (0.03 plant/ft2 [0.3/m2]) or 2 (0.01 plant/ft2 [0.1/m2]) (Ott et al. 2016).
Increasing the seeding depth reduced emergence of basalt milkvetch at two field sites in a dry spring and increased emergence at one site in a wet spring. The addition of row cover (N-sulate [DeWitt Co, Sikeston, MO]) increased emergence of basalt milkvetch at two sites. Emergence, however, was low overall in studies conducted at Fountain Green, Utah and Orovada and Wells, Nevada (Table 11, Jensen et al. 2022). Emergence of basalt milkvetch averaged 0.6% across sites, years, seeding depths, and row cover treatments. Timing and amount of spring precipitation had the greatest influence on seedling emergence for all 20 forb species evaluated. Odds of emergence were dramatically lower in 2014, which had a dry spring, than in 2013, which had a wet spring. Researchers hypothesized that seeding depth would correlate to seed size, but species, including the large-seeded basalt milkvetch, showed no clear pattern. Row cover, installed immediately after seeding and removed in mid-April, increased emergence of basalt milkvetch at Wells and Fountain Green. Soils were silt loams to loams in Wells, very fine sandy loams to silt loams in Orovada, and silty clay loams in Fountain Green. Forb species were seeded 0.5, 1, 1.4, and 1.6 in (1.4, 2.6, 3.6, and 4.0 cm) deep in late fall 2013 and again in fall 2014 using a tractor-drawn Hege seeder (Jensen et al. 2022).
Table 11. Effects of seeding depth (0.5-1.6 in) and use of row cover on first year emergence at two field sites in Nevada and one in Utah (Jensen et al. 2022).
Site | Orovada | Wells | Fountain Green | |||
Year | 2013 | 2014 | 2013 | 2014 | 2013 | 2014 |
Depth effects (% increase or decrease [-] in odds of emergence for a ~1 cm increase in seeding depth) | —- | —- | 10.7 | -77.5 | —- | -163.2 |
Mean emergence % (r = row cover, c = control) | —- | —- | 8.4 r 2.3 c |
0.25 r 0 c |
—- | 3.6 r 0.5 c |
No statistical differences reported.
—- indicates inadequate emergence response to quantify.
Fund et al. (2019) field tested many treatments to improve germination, emergence, establishment, and survival at three former agricultural sites in the eastern Great Basin (Downey, Idaho, and Clarkston and Spanish Fork, Utah). There was no combination of treatments that improved emergence and survival equally across sites. Acid scarification increased germination at all but the driest site, Spanish Fork. Seed coatings (fungicide [Obvius™] or fungicide + hydrophobic coatings) increased seedling emergence. Treatments to increase soil moisture had neutral or negative effects on seedling survival at all but the dry Spanish Fork site. Overall seedling survival to year 2 was low regardless of the combination of treatments, but researchers thought that earlier removal of seedling protection might have improved survival.
The treatments included seed pretreatments and site modifications to increase soil moisture (Fund et al. 2019). Seed pretreatments included no scarification, scarification, scarification + fungicide, scarification + hydrophobic coating, and scarification + fungicide + hydrophobic coating. Site modification treatments included controls, snow fencing to increase soil water availability, and plant protection fabric to limit predation and increase water availability. Snow fencing significantly increased snow depth over controls at Clarkston and Downey (P < 0.001) but not at Spanish Fork. Seeds used in this study were 91% viable. Seed was sown 0.4 to 0.8 in (1-2 cm) deep at a rate of 27 PLS/ft (90 PLS/m) of row. Because of the complexity of this study, it is described by major life stage in the paragraphs below.
Germination and seedling emergence were evaluated independently (Fund et al. 2019). Germination was evaluated through the burial of seeds in nylon mesh bags. Acid scarification increased germination at Downey (P = 0.0001) and Clarkston (P = 0.0033), but not at Spanish Fork. Fungicide or hydrophobic-only coatings did not increase germination compared with acid scarification at any site. At all sites, germination was significantly lower on snow fenced than control plots (P = 0.05) but was not different between controls and plant protection fabric or snow fencing and plant protection fabric.
Seedling Emergence was significantly increased with some seed pretreatments (Fund et al. 2019). Fungicide only or fungicide + hydrophobic coatings increased seedling emergence by an average of 37 to 112% relative to scarified seeds. At Downey, seedling emergence was highest in control plots (P = 0.01). At Clarkston, emergence was highest in fabric and control plots, which were not significantly different from one another. At Spanish Fork, emergence was highest in snow fenced and control plots, which were not significantly different from one another. Emergence of unscarified seed was lowest across all sites, but acid scarification did not result in increased seedling emergence compared to unscarified seed.
Seedling Establishment was highly variable (0.9-9%) at the end of the first growing season at all sites (Fund et al. 2019). At Downey and Clarkston, establishment was similar across site modification treatments. At Spanish Fork, establishment was highest in snow fenced plots (P = 0.05).
Survival to the second year was low at all sites with 6.25% of plants surviving at Downey, 0.06% at Clarkston, and 0.6% at Spanish Fork. At Spanish Fork, the driest site, survival was highest in snow fenced plots and significantly greater than fabric (P = 0.04) or control (P = 0.05) plots. Snow fencing had neutral or negative effects on survival at the other sites, and fabric had no positive effect at any life stage at any site. During this study spring precipitation was above, and summer precipitation was below the 30-year average. Researchers speculated that earlier removal of the plant protection fabric at sites where spring temperatures increased rapidly might improve seedling performance and survival (Fund et al. 2019).
Acknowledgements
Funding for Western Forbs: Biology, Ecology, and Use in Restoration was provided by the USDI BLM Great Basin Native Plant Materials Ecoregional Program through the Great Basin Fire Science Exchange. Great thanks to the chapter reviewers: Shaun Bushman, Plant Research Geneticist, USDA Agricultural Research Service and Arden Engel, Ecosystem Ecology Lab Manager, Boise State University.
This research was supported in part by the USDA Forest Service, Rocky Mountain Research Station. The findings and conclusions in this publication are those of the authors and should not be construed to represent any official USDA or U.S. Government determination or policy.
Literature Cited
Association of Official Seed Analysts [AOSA]. 2010. AOSA/SCST Tetrazolium testing handbook. Contribution No. 29. Lincoln, NE: Association of Official Seed Analysts.
Association of Official Seed Analysts [AOSA]. 2016. AOSA rules for testing seeds. Vol. 1. Principles and procedures. Washington, DC: Association of Official Seed Analysts.
Barneby, R.C. 1964. Atlas of North American Astragalus. Part I. The Phacoid and Homaloboid Astragali. & Part II. The Cercidothrix, Hypoglottis, Piptoloboid, Trimeniaeus and Orophaca Astragali. Memoirs of the New York Botanical Gardens. 13: 1-1188.
Barneby, R.C. 1989. Intermountain Flora Volume 3, Part B: Fabales. In: Cronquist, A.; Holmgren, A.H.; Holmgren, N.H.; Reveal, J.L.; Holmgren, P.K., eds. Intermountain flora: Vascular plants of the Intermountain West, U.S.A. Bronx, NY: The New York Botanical Garden. 279 p.
Basey, A.C.; Fant, J.B.; Kramer, A.T. 2015. Producing native plant materials for restoration: 10 rules to collect and maintain genetic diversity. Native Plants Journal. 16(1): 37-53.
Bates, J.D.; Miller, R.F.; Svejcar, T. 1998. Understory patterns in cut western juniper (Juniperus occidentalis spp. occidentalis Hook.) woodlands. The Great Basin Naturalist. 58(4): 363-374.
Bates, J.D.; Rhodes, E.C.; Davies, K. 2011. Impacts of fire on sage-grouse habitat and diet resources. Natural Resources and Environmental Issues. 17(5): 1-17.
Bates, J.D.; Svejcar, T.J. 2009. Herbaceous succession after burning of cut western juniper trees. Western North American Naturalist. 69(1): 9-25.
Bhattarai, K. 2007. Potential of basalt milkvetch (Astragalus filipes Torr. ex. A. Gray) populations and rhizobial strains for revegetation and restoration of Intermountain West rangelands. Logan, UT: Utah State University. 115 p.
Bhattarai, K.; Johnson, D.A.; Jones, T.A.; Connors, K.J.; Gardner, D.R. 2008. Physiological and morphological characterization of basalt milkvetch (Astragalus filipes): Basis for plant improvement. Rangeland Ecology and Management. 61(4): 444-455.
Bower, A.D.; St. Clair, J.B.; Erickson, V. 2014. Generalized provisional seed zones for native plants. Ecological Applications. 24(5): 913-919.
Bushman, B.S.; Bhattarai, K.; Johnson, D.A. 2010. Population structures of Astragalus filipes collections from western North America. Botany-Botanique. 88(6): 565-574.
Bushman, B.S.; Horning, M.E.; Shock, C.C.; Feibert, E.B.G.; Johnson, D.A. 2019. Dryland seedling emergence of basalt milkvetch (Astragalus filipes) and western prairie clover (Dalea ornata) under different planting seasons and seed treatments. Native Plants Journal. 20(3): 239-243.
Bushman, B.S.; Johnson, D.A.; Connors, K.J.; Jones, T.A. 2015. Germination and seedling emergence of three semiarid western North American legumes. Rangeland Ecology and Management. 68(6): 501-506.
Cane, J.H. 2008. Pollinating bees crucial to farming wildflower seed for U.S. habitat restoration. In: James, R.; Pitts-Singer, T., eds. Bees in agricultural ecosystems. Oxford, UK: Oxford University Press: 48-64.
Cane, J.H. 2011. Meeting wild bees’ needs on western US rangelands. Rangelands. 33(3): 27-32.
Cane, J.H.; Johnson, C.; Napoles, J.R.; Johnson, D.A.; Hammon, R. 2013. Seed-feeding beetles (Bruchinae, Curculionidae, Brentidae) from legumes (Dalea ornata, Astragalus filipes) and other forbs needed for restoring rangelands of the Intermountain West. Western North American Naturalist. 73(4): 477-484.
Cane, J.H.; Love, B. 2016. Floral guilds of bees in sagebrush steppe: Comparing bee usage of wildflowers available for postfire restoration. Natural Areas Journal. 36(4): 377-391.
Cane, J.H.; Love, B.; Swoboda, K. 2012. Breeding biology and bee guild of Douglas’ dustymaiden, Chaenactis douglasii (Asteraceae, Helenieae). Western North American Naturalist. 72(4): 563-568.
Consortium of Pacific Northwest Herbaria [CPNWH]. 2020. Seattle, WA: University of Washington Herbarium, Burke Museum of Natural History and Culture. http://www.pnwherbaria.org/index.php
de Graaff, M.A. 2016. Herbicide impacts on forb performance in degraded sagebrush steppe ecosystems. In: Kilkenny, F; Edwards, F.; Malcomb, A., eds. Great Basin Native Plant Project: 2015 Progress Report. Boise, ID: U.S. Department of Agriculture, Forest Service, Rocky Mountain Research Station: 218-228.
Eldredge, E.; Novak-Echenique, P.; Heater, T.; Mulder, A.; Jasmine, J. 2013. Plants for pollinator habitat in Nevada. Tech. Note NV 57. Reno, NV: U.S. Department of Agriculture, Natural Resources Conservation Service. 65 p.
European Native Seed Conservation Network [ENSCONET]. 2009. ENSCONET seed collecting manual for wild species. Edition 1: 32 p.
Fajemisin, B.; Ganskopp, D.; Cruz, R.; Vavra, M. 1996. Potential for woody plant control by Spanish goats in the sagebrush steppe. 20(3): 229-238.
Franklin, J.F.; Dyrness, C.T. 1973. Natural vegetation of Oregon and Washington. Gen. Tech. Rep. PNW-8. Portland, OR: U.S. Department of Agriculture, Forest Service, Pacific Northwest Forest and Range Experiment Station. 452 p.
Fund, A.J.; Hulvey, K.B.; Jensen, S.L.; Johnson, D.A.; Madsen, M.D.; Monaco, T.A.; Tilley, D.J.; Arora, E.; Teller, B. 2019. Basalt milkvetch responses to novel restoration treatments in the Great Basin. Rangeland Ecology and Management. 72(3): 492-500.
ITIS Database. 2023. Integrated Taxonomic Information System. Available: http://www.itis.gov/index.html
James, D.G.; Nunnallee, D. 2011. Life histories of Cascadia butterflies. Corvallis, OR: Oregon State University Press. 447 p.
Jensen, S.; Christensen, W.F.; Roundy, B.; Anderson, V.J.; Kitchen, S.G.; Allphin, L. 2022. Temporal and spatial factors influence native forb emergence more than sowing depth. Rangeland Ecology and Management. 83: 41-49.
Johnson, C.G.; Swanson, D.K. 2005. Bunchgrass plant communities of the Blue and Ochoco Mountains: A guide for managers. R6-NR-ECOL-TP-06-98. Portland, OR: U.S. Department of Agriculture, Forest Service, Pacific Northwest Research Station. 128 p.
Johnson, D.A.; Jones, T.A.; Bhattarai, K.; Connors, K.J.; Bushman, B.S.; Jensen, K.B. 2008. Notice of release of NBR-1 Germplasm basalt milkvetch. Native Plants Journal. 9(2): 127-132.
Jones, T.A.; Johnson, D.A.; Bushman, B.S.; Connors, K.J.; Smith, R.C. 2016. Seed dormancy mechanisms in basalt milkvetch and western prairie clover. Rangeland Ecology and Management. 69(2): 117-122.
Kildisheva, O.A.; Erickson, T.E.; Madsen, M.D.; Dixon, K.W.; Merritt, D.J. 2018a. Seed germination and dormancy traits of forbs and shrubs important for restoration of North American dryland ecosystems. Plant Biology. 21(2019): 458-469.
Kildisheva, O.A.; Erickson, T.E.; Merritt, D.J.; Madsen, M.D.; Dixon, K.W.; Vargas, J.; Amarteifio, R.; Kramer, A.T. 2018. Do abrasion- or temperature-based techniques more effectively relieve physical dormancy in seeds of cold desert perennials? Rangeland Ecology and Management. 71(3): 318-322.
Kratsch, H.A.; Johnson, D.; Connors, K. 2010. Demonstration, education, and outreach activities related to GBNPSIP Plant Materials. In: Shaw, N.L.; Pellant, M., eds. Great Basin Native Plant Selection and Increase Project: 2009 Progress Report. Boise, ID: U.S. Department of Agriculture, Forest Service, Rocky Mountain Research Station: 25-32.
Lambert, S. 2005. Guidebook to the seeds of native and non-native grasses, forbs and shrubs of the Great Basin. Boise, ID: U.S. Department of the Interior, Bureau of Land Management, Idaho State Office. 136 p.
Love, B.; Cane, J. 2019. Mortality and flowering of Great Basin perennial forbs after experimental burning: Implications for wild bees. Rangeland Ecology and Management. 72(2): 310-317.
Luna, T.; Mousseaux, M.R.; Dumroese, R.K. 2018. Common native forbs of the northern Great Basin important for greater sage-grouse. Gen. Tech. Rep. RMRS-GTR-387. Fort Collins, CO: U.S. Department of Agriculture, Forest Service, Rocky Mountain Research Station; Portland, OR: U.S. Department of the Interior, Bureau of Land Management, Oregon-Washington Region. 76 p.
Mansfield, D.H. 2000. Flora of Steens Mountain. Corvallis, OR: Oregon State University Press. 410 p.
Marlette, G.M.; Anderson, J.E. 1986. Seed banks and propagule dispersal in crested-wheatgrass stands. Journal of Applied Ecology. 23(1): 161-175.
Martin, A.C.; Zim, H.S.; Nelson, A.L. 1951. American wildlife and plants: A guide to wildlife food habits. New York, NY: Dover Publications. 500 p.
Miller, R.F.; Svejcar, T.J.; Rose, J.A. 2000. Impacts of western juniper on plant community composition and structure. Journal of Range Management. 53(6): 574-585.
Mohan, S.K.; Shock, C.C. 2014. Etiology, epidemiology, and management of diseases of native wildflower seed production. In: Kilkenny, F.; Shaw, N.L.; Gucker, C.L., eds. Great Basin Native Plant Project: 2013 Progress Report. Boise, ID: U.S. Department of Agriculture, Forest Service, Rocky Mountain Research Station: 107-108.
Munz, P.A.; Keck, D.D. 1973. A California flora and supplement. Berkeley, CA: University of California Press. 1905 p.
Ogle, D.; Pavek, P.; Fleenor, R.; Stannard, M.; Dring, T.; Cane, J.; Fullen, K.; St. John, L.; Tilley, D. 2011. Plants for pollinators in the Inland Northwest. Tech. Note 2B. Boise, ID: U.S. Department of Agriculture, Natural Resources Conservation Service. 65 p.
Ogle, D.; St. John, L.; Stannard, M.; Holzworth, L. 2012. Conservation plant species for the Intermountain West. Plant Materials Technical Note 24. Boise, ID: U.S. Department of Agriculture, Natural Resources Conservation Service. 57 p.
Ogle, D.; Tilley, D.; Cane, J.; St. John, L.; Fullen, K.; Stannard, M.; Pavek, P. 2019. Plants for pollinators in the Intermountain West. Plant Materials Tech. Note 2A. Boise, ID: U.S. Department of Agriculture, Natural Resources Conservation Service. 40 p.
Omernik, J.M. 1987. Ecoregions of the conterminous United States. Map (scale 1:7,500,000). Annals of the Association of American Geographers. 77(1): 118-125.
Ott, J.E.; Cox, R.D.; Shaw, N.L.; Newingham, B.A.; Ganguli, A.C.; Pellant, M.; Roundy, B.A.; Eggett, D.L. 2016a. Post-fire drill-seeding of Great Basin plants: Effects of contrasting drills on seeded and nonseeded species. Rangeland Ecology & Management. 69(5): 373-385.
Pavek, P.; Erhardt, B.; Heekin, T.; Old, R. 2012. Forb seedling identification guide for the Inland Northwest: Native, introduced, invasive, and noxious species. Pullman, WA: U.S. Department of Agriculture, Natural Resources Conservation Service, Pullman Plant Materials Center. 144 p.
Pennington, V.E.; Schlaepfer, D.R.; Beck, J.L.; Bradford, J.B.; Palmquist, K.A.; Lauenroth, W.K. 2016. Sagebrush, greater sage-grouse, and the occurrence and importance of forbs. Western North American Naturalist. 76(3): 298-312.
Plant Conservation Alliance [PCA]. 2015. National seed strategy for rehabilitation and restoration 2015-2020. Washington, DC: U.S. Department of the Interior, Bureau of Land Management. 52 p.
Plummer, A.P. 1977. Revegetation of disturbed Intermountain area sites. In: Thames, J.L., ed. Reclamation and use of disturbed land in the southwest. Tucson, AZ: University of Arizona: 302-339.
Pyle, W.H. 1993. Response of brood-rearing habitat of sage grouse to prescribed burning in Oregon. Corvallis, OR: Oregon State University. Thesis. 56 p.
Ralphs, M.H.; Creamer, R.; Baucom, D.; Gardner, D.R.; Welsh, S.L.; Graham, J.D.; Hart, C.; Cook, D.; Stegelmeier, B.L. 2008. Relationship between the endophyte Embellisia spp. and the toxic alkaloid swainsonine in major locoweed species (Astragalus and Oxytropis). Journal of Chemical Ecology. 34(1): 32-38.
Ransom, C. 2014. Evaluation of imazapic rates and forb planting times on native forb establishment. In: Kilkenny, F.; Shaw, N.; Gucker, C., eds. Great Basin Native Plant Project: 2013 Progress Report. Boise, ID: U.S. Department of Agriculture, Forest Service, Rocky Mountain Research Station: 191-196.
Ransom, C.; Edvarchuk, K. 2009. Developing strategies for selective herbicide use in native forb seed production. In: Shaw, N.; Pellant, M., eds. Great Basin Native Plant Project: 2008 Progress Report. Boise, ID: U.S. Department of Agriculture, Forest Service, Rocky Mountain Research Station: 122-125.
Richardson, B.; Kilkenny, F.; St. Clair, B.; Stevenson-Molnar, N. 2020. Climate Smart Restoration Tool. https://climaterestorationtool.org/csrt/
Sanderson, M.J. 1991. Phylogenetic relationships within North American Astragalus L. (Fabaceae). Systematic Botany. 16(3): 414–430.
Sapsis, D.B. 1990. Ecological effects of spring and fall prescribed burning on basin big sagebrush/Idaho fescue-bluebunch wheatgrass communities. Corvallis, OR: Oregon State University. Thesis. 106 p.
SEINet – Regional Networks of North American Herbaria Steering Committee [SEINet]. 2020. SEINet Regional Networks of North American Herbaria. https://Symbiota.org/docs/seinet
Shock, C.C.; Feibert, E.B.G.; Rivera, A.; Saunders, L.D.; Johnson, D.A.; Bushman, S.; Shaw, N.; Kilkenny, F. 2018a. Prairie clover and basalt milkvetch seed production in response to irrigation. In: Kilkenny, F; Edwards, F.; Irwin, J.; Barga, S., eds. Great Basin Native Plant Project: 2017 Progress Report. Boise, ID: U.S. Department of Agriculture, Forest Service, Rocky Mountain Research Station: 142-148.
Shock, C.C.; Feibert, E.B.G.; Rivera, A.; Shaw, N.; Kilkenny, F.F. 2018b. Irrigation requirements for seed production of three leguminous wildflowers of the U.S. Intermountain West. HortScience. 53(5): 692-697.
Shock, C.C.; Feibert, E.B.G.; Saunders, L.D.; Parris, C.; Shaw, N.; Johnson, D.A.; Bushman, B.S.; Sampangi, R.K. 2013. Direct surface seeding strategies for the establishment of two native legumes of the Intermountain West. In: Kilkenny, F; Shaw, N.; Gucker, C., eds. Great Basin Native Plant Project: 2012 Progress Report. Boise, ID: U.S. Department of Agriculture, Forest Service, Rocky Mountain Research Station: 140-144.
Shock, C.C.; Feibert, E.B.G.; Saunders, L.D.; Parris, C.; Shaw, N.; Sampangi, R. 2011. Seed production of Great Basin native forbs- Subsurface drip irrigation (SDI) for stable, efficient native forb seed production using small amounts of supplemental irrigation water; weed control; and seeding practices. In: Shaw, N.; Pellant, M., eds. Great Basin Native Plant Selection and Increase Project: 2010 Progress Report. Boise, ID: U.S. Department of Agriculture, Forest Service, Rocky Mountain Research Station: 93-128.
Shock, C.C.; Feibert, E.B.G.; Saunders, L.D.; Shaw, N. 2014. Irrigation requirements for native wildflower seed production for perennial and annual species planted in fall of 2009. In: Kilkenny, F; Shaw, N.; Gucker, C., eds. Great Basin Native Plant Project: 2013 Progress Report. Boise, ID: U.S. Department of Agriculture, Forest Service, Rocky Mountain Research Station: 134-141.
Society for Ecological Restoration; International Network for Seed Based Restoration; Royal Botanic Gardens [SER, INSR, RBGK]. 2023. Seed Information Database (SID). Available from: https://ser-sid.org/
Spellenberg, R. 1976. Chromosome numbers and their cytotaxonomic significance for North American Astragalus (Fabaceae). Taxon. 25(4): 463-476.
Tilley, D.; Fund, A.; Pickett, T. 2017. Plant guide for basalt milkvetch (Astragalus filipes Torr. ex A. Gray). Aberdeen, ID: U.S. Department of Agriculture, Natural Resources Conservation Service. 4 p.
Tilley, D.; Ogle, D. 2011. Using historic bushel weights to estimate seed quality. Native Plants Journal. 12(2): 107-110.
Turner, N.J.; Thompson, L.C.; Thompson, M.T.; York, A.Z. 1990. Thompson ethnobotany: Knowledge and usage of plants by the Thompson Indians of British Columbia. Royal British Columbia Museum Memoir No. 3. Victoria, British Columbia, Canada: Royal British Columbia Museum. 335 p.
USDA Forest Service, Bend Seed Extractory [USDA FS BSE]. 2017. Nursery Management Information System Version 4.1.11. Local Source Report 34-Source Received. Bend, OR: U.S. Department of Agriculture, Forest Service, Bend Seed Extractory.
USDA Forest Service, Western Wildland Environmental Threat Assessment Center [USFS WWETAC]. 2017. TRM Seed Zone Applications. Prineville, OR: U.S. Department of Agriculture, Forest Service, Western Wildland Environmental Threat Assessment Center. https://www.fs.fed.us/wwetac/threat-map/TRMSeedZoneMapper.php
USDA Natural Resources Conservation Service [USDA NRCS]. 2023. The PLANTS Database. Greensboro, NC: U.S. Department of Agriculture, Natural Resources Conservation Service, National Plant Data Team. https://plants.usda.gov/java
USDI Bureau of Land Management, Seeds of Success [USDI BLM SOS]. 2017. Seeds of Success collection data. Washington, DC: U.S. Department of the Interior, Bureau of Land Management, Plant Conservation and Restoration Program.
USDI Bureau of Land Management, Seeds of Success [USDI BLM SOS]. 2021. Bureau of Land Management technical protocol for the collection, study, and conservation of seeds from native plant species for Seeds of Success. Washington, DC: U.S. Department of the Interior, Bureau of Land Management, Plant Conservation and Restoration Program. 44 p.
USDI Environmental Protection Agency [USDI EPA]. 2017. Ecoregions. Washington, DC: U.S. Department of the Interior, Environmental Protection Agency. https://www.epa.gov/eco-research/ecoregions
USDI Geological Survey [USDI USGS]. 2020. Biodiversity Information Serving Our Nation (BISON). U.S. Geological Survey. https://www.gbif.us/#home
Utah Crop Improvement Association [UCIA]. 2015. How to be a seed connoisseur. Logan, UT: UCIA, Utah Department of Agriculture and Food, Utah State University and Utah State Seed Laboratory. 16 p.
Waichler, W.S.; Miller, R.F.; Doescher, P.S. 2001. Community characteristics of old-growth western juniper woodlands. Journal of Range Management. 54(5): 518-527.
Watrous, K.M. 2010. Reproductive ecology of Astragalus filipes, a Great Basin restoration legume. Logan, UT: Utah State University. Thesis. 57 p.
Watrous, K.M.; Cane, J.H. 2011. Breeding biology of the threadstalk milkvetch, Astragalus filipes (Fabaceae), with a review of the genus. American Midland Naturalist. 165(2): 225-240.
Welsh, S.L.; Atwood, N.D.; Goodrich, S.; Higgins, L.C., eds. 2015. A Utah Flora. Fifth Edition, revised. Provo, UT: Brigham Young University. 990 p.
Young, S.A.; Schrumpf, B.; Bouck, M.; Moore, M. 2020. How the Association of Official Seed Certifying Agencies (AOSCA) tracks wildland sourced seed and other plant propagating materials. Logan, UT: Utah State University, Utah Agricultural Experiment Station. 36 p.
Youtie, B.A.; Miller, R.F. 1986. Insect predation on Astragalus filipes and Astragalus purshii seeds. Northwest Science. 60(1): 42-46.
Zimmers, J.C.; Thomas, M.; Yang, L.; Bombarely, A.; Mancuso, M.M.; Wojciechowski, M.F.; Smith, J.F. 2017. Species boundaries in the Astragalus cusickii complex delimited using molecular phylogenetic techniques. Molecular Phylogenetics and Evolution. 114: 93-110.
How to Cite
Gucker, C.L.; Shaw, N.L. 2023. Basalt milkvetch (Astragalus filipes [Torr. ex. A. Gray] Raf.). In: Gucker, C.L.; Shaw, N.L., eds. Western forbs: Biology, ecology, and use in restoration. Reno, NV: Great Basin Fire Science Exchange. https://westernforbs.org/species/basalt-milkvetch-astragalus-filipes/