Authorship
Gucker, Corey; Shaw, Nancy
Publication Date
March 2018
Update
May 2024
Nomenclature
Sphaeralcea grossulariifolia (Hook. & Arn.) Rydb., hereafter referred to as gooseberryleaf globemallow, belongs to the Malvoideae subfamily of the Malvaceae or mallow family (Kearney 1935; La Duke 2016).
Family
Malvaceae – Mallow family
Genus
Sphaeralcea
Species
grossulariifolia
NRCS Plant Code
SPGR2 (USDA NRCS 2024).
Subtaxa
The Flora of North America (La Duke 2016) does not recognize any varieties or subspecies.
Synonyms
Malvastrum coccineum (Nuttall) A. Gray var. grossulariifolium (Hooker & Arnott) Torrey, M. grossulariifolium (Hooker & Arnott) A. Gray, Sida grossulariifolia Hooker & Arnott, Sphaeralcea grossulariifolia subsp. pedata (Torrey ex A. Gray) Kearney, S. grossulariifolia var. pedata (Torrey ex A. Gray) Kearney, S. pedata Torrey ex A. Gray (La Duke 2016).
Common Names
Gooseberryleaf globemallow, current-leaf globemallow (La Duke 2016; LBJWC 2023; ITIS 2024).
Chromosome Number
Chromosome number is stable, 2n = 20, and plants are diploid (La Duke 2016).
Hybridization
Hybridization occurs within the Sphaeralcea genus. Gooseberryleaf globemallow hybridizes with scarlet globemallow (S. coccinea), small-leaf globemallow (S. parvifolia), Munro’s globemallow (S. munroana), and Rusby’s globemallow (S. rusbyi) (Atwood and Welsh 2002; Welsh et al. 2015). The resulting intermediate hybrids make identification challenging.
Distribution
Gooseberryleaf globemallow is native to North America and occurs primarily in the western states of Washington, Oregon, Idaho, Nevada, Utah, California, Arizona, and New Mexico (La Duke 2016). It may reach its maximum abundance in Utah (Kearney 1935). Plants are winter hardy, especially in regions with at least periodic snow cover. They are most abundant on open or disturbed sites receiving 8 to 12 in (200–300 mm) of annual precipitation (Wasser 1982; Stevens et al. 1985).
Habitat And Plant Associations
Gooseberryleaf globemallow is common and widespread in salt, warm, and cool desert shrublands (Fig. 1). It also occurs in openings of mountain brush communities, pinyon-juniper (Pinus–Juniperus spp.) woodlands, and ponderosa pine (P. ponderosa) forests (Blaisdell and Holmgren 1984; Atwood and Welsh 2002; Stevens and Monsen 2004b; Welsh et al. 2015). Common vegetation associates are shadscale (Atriplex confertifolia), big sagebrush (Artemisia tridentata), blackbrush (Coleogyne ramosissima), and rabbitbrush (Chrysothamnus and Ericameria spp.) (Flowers 1934; Fautin 1946; Plummer et al. 1968; Horton 1989; Donart 1994; Alzerreca-Angelo et al. 1998; Stevens and Monsen 2004b).
Figure 1. Gooseberryleaf globemallow growing on Milford Flat, Milford County, UT. Photo: S. Young, Utah State University (USU).
Elevation
Gooseberryleaf globemallow occupies sites at elevations from 330 to 7,500 ft (100–2,300 m) across its range (Blackwell 2006; La Duke 2016; UW Extension 2024). In Utah it is found at elevations ranging from 2,600 to 7,500 ft (790-2,290 m) (Welsh et al. 2015).
Soils
Plants are drought tolerant and grow on most soil textures, but are common on volcanic substrates. Sandy to clay loams are common habitats, but plants also occur on rocky or gravelly soils and shallow foothills soils (Wasser 1982; Belcher 1985; Pendery and Rumbaugh 1986; La Duke 2016). Plants are most frequent on basic soils (Kearney 1935; Horton 1989; Pendery and Rumbaugh 1993). Moderately saline soils are tolerated but sodic soils are not (Wasser 1982).
In a greenhouse study evaluating growth in field-collected soils, emergence and short-term survival were significantly greater (P < 0.05) in sandy loam than in clay loam soils. Sandy loam soils were 69% sand, 13% clay, and 19% silt; clay loams were 35% sand, 30% clay, and 36% silt (Rawlins et al. 2009).
Description
Gooseberryleaf globemallow is a morphologically diverse perennial forb (Kearney 1935; Stevens and Monsen 2004b; Tilley et al. 2011; La Duke 2016). Many intermediate forms are possible where its distribution overlaps with scarlet, small-leaf, Rusby’s, or Munro’s globemallow, with which it freely hybridizes (Atwood and Welsh 2002; Tilley et al. 2011; Sriladda et al. 2012; Welsh et al. 2015).
Plants produce a large, deep, branching taproot with several surface feeding roots (Kearney 1935; Wasser 1982; Mee et al. 2003; Holmgren et al. 2005; Tilley et al. 2011). Multiple erect to somewhat spreading stems develop from the branching woody caudex (Mee et al. 2003; Holmgren et al. 2005; Pavek et al. 2012; Welsh et al. 2015). Stems are few to many in loose tufts and typically measure 24 to 39 in (60–100 cm) tall, but height varies greatly (Wasser 1982; La Duke 2016). Stems are up to 4 mm in diameter at the base (Kearney 1935) and range from hairless to sparsely stellate to densely covered with short, fine, white hairs and thus are green to gray-green, yellowish, or whitish (Atwood and Welsh 2002; Welsh et al. 2015; La Duke 2016). Density of hairs may decrease as stems age (Kearney 1935; Holmgren et al. 2005).
Leaves are alternate and resemble those of gooseberries (Ribes spp.) . Petioles of lower leaves are up to 2.5 in (6.3 cm) long, but shorter on upper leaves. Leaves are typically triangular with three to five lobes that are often divided nearly to the midvein (Parkinson 2003; Holmgren et al. 2005; Welsh et al. 2015; La Duke 2016), but sometimes lobed, crenate, or again divided (Blackwell 2006; Goodrich and Neese 1986). Veins are most prominent near the leaf bases (Kearney 1935). Leaves are often thin and 0.4 to 2 in (1.3–5 cm) long, although lengths of 3.5 in (9 cm) have been reported. Leaves are generally slightly longer than wide (Mee et al. 2003; Holmgren et al. 2005; Welsh et al. 2015; La Duke 2016). Teeth on the leaf margins are irregularly pointed to rounded (Kearney 1935; Wasser 1982; La Duke 2016). Herbage color is highly variable, ranging from white or gray to yellow or green depending on the presence and density of stellate hairs (Welsh et al. 2015).
Flowers are usually two to several per node on an elongate, narrow, interrupted thyrsoid panicle (Fig. 2) (Mee et al. 2003; Welsh et al. 2015). The calyx is bell shaped with ovate to lance-shaped sepals covered with stellate hairs. Petals are five, red to red-orange or reddish-pink, and 0.3 to 0.7 in (8-18 mm) long and exceed the sepals. Stamens are numerous and coalescent, forming a tube (the staminal column). Anthers are yellow (Wasser 1982; Atwood and Welsh 2002; Holmgren et al. 2005; Pavek et al. 2012; Welsh et al. 2015; La Duke 2016). Fruits are densely pubescent hemispheric schizocarps, each consisting of 10 to 12 one or two-seeded mericarps (carpels) arranged in a ring (Kearney 1935; Wasser 1982; Belcher 1985; La Duke 2016). Schizocarps measure 2.5 to 3.5 mm long and are slightly less wide (2–2.5 mm) (Figs. 3 and 4). Mericarps dehisce along the upper one-third to one half, which is papery and veiny, exposing the seeds. The lower portion is reticulate and indehiscent (Holmgren et al. 2005; Welsh et al. 2015; La Duke 2016; Hitchcock and Cronquist 2018). Seeds are gray to black, kidney shaped, glabrous or covered with short, soft hairs, and contain little or no endosperm. Seeds often have the lacy fruit coat attached when the mericarps dehisce naturally (Fig. 4) (Belcher 1985).
Figure 2. Gooseberryleaf globemallow flowers. Photo: USDI Bureau of Land Management (BLM) ID931 Seeds of Success (SOS).
The mericarp is the structure that is dispersed at reproductive maturity. This structure naturally dehisces but unevenly over time. For the remainder of this chapter, the term seed will refer to both the mericarp and its seeds.
Figure 3. Gooseberryleaf globemallow schizocarps (whole fruits), mericarps (segments of the schizocarps), and individual seeds (black). Photo: BLM NM930 SOS.
Figure 4. Gooseberryleaf globemallow seeds (scale is cm). Photo: BLM ID931 SOS.
Reproduction
Plants reach reproductive maturity by their second year (Wasser 1982), and flowering is episodic and extensive (Sharp et al. 1990; Kitchen 1994). Many researchers have reported reproductive explosions and subsequent disappearance of plants (Shreve and Hinckley 1937; Blaisdell and Holmgren 1984; Sharp et al. 1990; Kitchen 1994). Kitchen (1994) concluded that favorable germination conditions trigger explosive reproduction. Blaisdell and Holmgren (1984) indicated that favorable establishment conditions are required every 2 to 3 years for the species to persist on a site. Shreve and Hinckley (1937) noted that plants disappeared and reappeared during periodic visits to desert sites near Tucson, Arizona. While no research has specifically investigated the origin of plants in a year of extensive flowering, the appearance of many large, seemingly mature plants following a year of relative absence, suggests that boom populations may be a mixture of seed germination and sprouting from dormant plants (S. Young, Utah State University [USU]-retired, personal communication, February 2018).
While the episodic, boom and bust reproduction suggests gooseberryleaf globemallow is short-lived, a very small percentage of plants may be long-lived. On the Desert Experimental Range in southwestern Utah, West (1979) followed a seedling cohort and found a small number of plants lived for 33 years in the salt desert shrub community (West 1979).
Repeat photo monitoring suggests that the timing and amount of spring precipitation may be important for episodic explosions of flowering and reproduction. Photo monitoring was conducted over a 40-year time frame in a shadscale shrubland in the Raft River Valley of south-central Idaho. The largest gooseberryleaf globemallow floral display occurred in 1964 when spring precipitation was three times the average. Plants were also present and flowered, but much less in years when spring precipitation was below average (Sharp et al. 1990).
Figure 5. Gooseberry globemallow flower boom on the Colorado Plateau. Photo: BLM CP2 SOS.
Phenology
Gooseberryleaf globemallow flowers indeterminately. While flowers are typical in spring or summer and generally most abundant from May through July (Stevens et al. 1985; La Duke 2016), flowering can continue into September or later (Shock et al. 2015; USDI BLM SOS 2017, 2024). Seed is typically mature in June, July, or August (Stevens et al. 1985).
Breeding System
Gooseberryleaf globemallow is strongly outcrossing and perhaps even self-sterile (Kearney 1935).
Pollination
Gooseberryleaf globemallow is insect-pollinated and especially attractive to bees (Pendery and Rumbaugh 1986; Ogle et al. 2011; Tepedino 2017). The globemallow bee (Diadasia diminuta) is a globemallow (Sphaeralcea spp.) specialist and an important pollinator for gooseberryleaf globemallow (Tepedino 2017). Other bees (Apis, Agapostemon, Calliopsis, Halictus, and Melissodes spp.) and wasps (Ammoplanus spp.) are also important globemallow pollinators (Pendery and Rumbaugh 1986). At sites in Idaho, Nevada, Utah, and Wyoming, an average of 14.5 native bee visitors were observed for every 100 globemallow (gooseberryleaf and Munro’s) plants examined (Cane and Love 2016).
Ecology
Gooseberryleaf globemallow is frequently associated with disturbed sites, but it is also a common component of late-seral shrubland and woodland vegetation (Sharp et al. 1990; Stevens and Monsen 2004a, b).
Seed And Seedling Ecology
Partially dehiscent gooseberryleaf globemallow fruits are considered an adaptation to exploit a range of dispersal conditions and increase the chances of capitalizing on favorable germination conditions. Dehiscent portions of the mericarp release the seed as soon as they are mature, while the indehiscent portion holds seeds until they are dislodged or later when natural dehiscence occurs after a period of weeks or months (Kearney 1935). Colonization of disturbed sites is primarily thought to be from germination of soil-stored seed (Plummer 1958; Wasser 1982; Ott et al. 2001).
Disturbance Ecology
Abundance of gooseberryleaf globemallow is often greater in open or recently disturbed than in undisturbed sites (Plummer 1958; Wasser 1982; Ott et al. 2001). Plants were either restricted to or had greater abundance on mechanically disturbed than undisturbed sites in Utah. Growth was “remarkable” by the third year after cabling a Utah juniper-singleleaf pinyon (J. osteosperma–P. monophylla) woodland where it was inconspicuous before treatment and on undisturbed sites (Plummer 1958). In Wyoming big sagebrush (Artemisia tridentata subsp. wyomingensis) and Utah juniper communities, established gooseberryleaf globemallow plants were relatively unaffected by chaining that followed post-fire aerial seeding (Ott et al. 2003).
Fire response. Gooseberryleaf globemallow is common on burned sites and its abundance is often greater on burned than on unburned sites. Plants were absent from unburned sites but occurred on 2-, 6-, and 17-year-old burned blackbrush-dominated sites in southwestern Utah. Cover was greatest on 6-year-old burned plots (Callison et al. 1985). In west-central Utah, frequency was slightly greater on burned than unburned sites visited 1, 2, and 3 years after a summer wildfire that killed nearly all nonsprouting perennials in big sagebrush and Utah juniper vegetation (Ott et al. 2001). In central Arizona, production of gooseberryleaf globemallow increased after fire in lovegrass (Eragrostis spp.)-dominated grasslands even when post-fire growing season precipitation was about 7 in (180 mm) below normal. On north slopes, gooseberryleaf globemallow production was 9 lbs/ac (10 kg/ha) before the fire and 30 lbs/ac (34 kg/ha) in the first post-fire growing season. On south slopes, production was 10 lbs/ac (11 kg/ha) before and 18 lbs/ac (20 kg/ha) after the fire (Pase and Knipe 1977).
Love and Cane (2019) examined survival and post-burning flowering responses of six common Great Basin forbs to a realistic range of fire severities using a calibrated burn barrel. Burn treatments of selected intensities and duration (Table 1) were applied to plants growing in study plot rows at Oregon State University’s Malheur Experiment Station near Ontario, Oregon, in late August as plants were entering senescence. Gooseberryleaf globemallow was ranked as fire intolerant. Its mortality was less than 40% for the very low, low, and medium treatments. Mortality from the high treatment was just over 40% and near 60% for the very high treatment.
Table 1. Burn prescriptions and associated peak temperatures at the soil surface and at a 2-cm depth (Love and Cane 2019).
Treatment | Prescription | Peak surface temperature (°F) | Peak temperature 0.8 in depth (°F) |
Control | No fuel | Ambient | Ambient |
Very Low | 52 kPa (7.5 psi), 25 sec | 270 ± 67 | 120 ± 7 |
Low | 52 kPa (7.5 psi), 50 sec | 466 ± 108 | 135 ± 7 |
Medium | 103 kPa (15 psi), 65 sec | 667 ± 56 | 151 ± 11 |
High | 103 kPa (15 psi), 175 sec | 932 ± 140 | 216 ± 41 |
Very High | 103 kPa (15 psi), 240 sec | 1,164 ± 94 | 264 ± 27 |
Land-use response. Land-use legacies may also affect abundance of gooseberryleaf globemallow (Morris et al. 2013). When abandoned old-fields (dryland farmed in the 1910s) were compared to uncultivated sagebrush vegetation in northwestern Utah in 2011, the biomass of gooseberryleaf globemallow was about half as much in the old fields. In a follow-up greenhouse study, survivorship was significantly (P = 0.01) less when gooseberryleaf globemallow seeds were grown in soil collected from the old field (73%) than from the non-cultivated (100%) site. Survivorship and growth differences could not be linked to soil nutrient differences, which suggested other abiotic factors may affect plant performance in old fields and non-cultivated sites (Morris et al. 2013).
Grazing response. Although described as grazing tolerant, abundance of gooseberryleaf globemallow was often less on sheep-grazed than ungrazed sites, regardless of the season of use. Close grazing or grazing after prolonged drought often resulted in plant injury or loss (Pendery and Rumbaugh 1986; Stevens and Monsen 2004b). When long-term (59 yrs) effects of winter and spring sheep grazing were compared in exclosures and grazed sites at Utah’s Desert Experimental Range, frequency was lower on grazed than exclosure plots, but not significantly so (Kitchen and Hall 1996). In a newly established pasture near Kimberly, Idaho, mortality of gooseberryleaf globemallow was greater in plots grazed by sheep in fall (43%) than in ungrazed plots (24%). While mortality differences may have been exacerbated by a clipping treatment conducted in the first year of the study, mortality was also greater in grazed than ungrazed plots where clipping did not occur (Rumbaugh et al. 1993a). West (1979) found seedling establishment and persistence were less on winter sheep-grazed plots than on ungrazed plots in a salt desert shrubland at Utah’s Desert Experiment Range (Table 2).
Table 2. Establishment and survival of 1935 seedling cohorts of gooseberryleaf globemallow with and without winter sheep grazing. Total number of seedlings surviving on plots (4 each, plot size: 5 × 20 ft) at the Desert Experimental Range in Millard County, UT (West 1979).
Year |
1935 (est. yr) |
1936 |
1937 |
1958 |
1968 |
————seedling no.———— |
|||||
Grazed |
51 |
36 |
28 |
5 |
4 |
Ungrazed |
189 |
111 |
91 |
6 |
5 |
Ingested seed may be dispersed by livestock (Whitacre and Call 2006). In a controlled study, about 50% of the seeds fed to heifers were recovered within the first 3 days of ingestion. Seed recovery was greatest 1 day after ingestion (28%), and increased time in the gut decreased seed germinability. Germination was significantly greater (17.7%) after 1 day than after 2 days (8.7%) or 3 days (10.4%) in the gut (P ≤ 0.05). Germination of control seed was 40% (Whitacre and Call 2006).
Wildlife And Livestock Use
Gooseberryleaf globemallow is widely utilized by wildlife and livestock (Ogle et al. 2011). Plants are green early in the spring (mid- or late March) and will green up again in late September or October with seasonal storms (Horton 1989; Monsen and Stevens 2004; Ogle et al. 2012). Deer (Odocoileus spp.), elk (Cervus canadensis), pronghorn (Antilocapra americana), and bighorn sheep (Ovis canadensis) feed on the forage, and birds and rabbits feed on the seed (Hancock 1966; Kufeld 1973; Kufeld et al. 1973; Wasser 1982). Globemallow species are also considered a preferred greater sage-grouse (Centrocercus urophasianus) food (Dumroese et al. 2016).
Plants are considered most palatable to pronghorn when flowering (Pendery and Rumbaugh 1986). On the Desert Experimental Range in western Utah, globemallows (gooseberryleaf was most common) were preferred by pronghorn (Beale and Smith 1970). Globemallow use was high in spring (March-May), wet summers (June-August), and wet falls (September-November). In an uncharacteristically wet June and July, globemallow made up 34% of pronghorn diets. The considerable drought tolerance of globemallows provided green forage in most summers (Beale and Smith 1970).
Utilization of gooseberryleaf globemallow by domestic sheep was high on spring- and winter-grazed sites in Idaho and Utah. Average utilization was 80% in fall and winter after 10 years of evaluation of winter sheep range in west-central Utah (Hutchings and Stewart 1953). Average sheep consumption was 70% in spring and 63% in fall after 2 years in newly transplanted pastures of crested wheatgrass (Agropyron cristatum), alfalfa (Medicago sativa), and gooseberryleaf globemallow near Kimberly, Idaho (Rumbaugh et al. 1993a).
Nutritional Value
Gooseberryleaf globemallow meets the dietary requirements of sheep and cattle (Pendery and Rumbaugh 1993). In studies of spring nutrition, in vitro digestibility averaged 70% and crude protein averaged 20% (Welch 2004). Forage characteristics of gooseberry globemallow in new pastures near Kimberly, Idaho (Table 3), met the nutritive requirements for sheep and medium-sized, yearling heifers (Rumbaugh et al. 1993b).
Table 3. Nutritional content (fall and spring concentrations averaged) of gooseberryleaf globemallow plants growing near Kimberly, Idaho (Rumbaugh et al. 1993b).
Measurement |
Microminerals (µg/g) |
||||
Element |
Cu |
Fe |
Mn |
Na |
Zn |
Leaves |
14 |
1560 |
62 |
160 |
41 |
Stems |
15 |
390 |
22 |
320 |
33 |
Measurement |
Macrominerals (mg/g) |
||||
Element |
Ca |
Mg |
N |
P |
K |
Leaves |
24 |
5.4 |
38 |
3.9 |
18 |
Stems |
14 |
4.5 |
23 |
2.3 |
20 |
Ethnobotany
The medicinal value of gooseberryleaf globemallow may be limited. The Hopi used it to ease consitipation and treat broken bones. The also mixed parts of the plant with clay when making pottery (Moerman 2003).
Horticulture
Gooseberryleaf globemallow is grown and sold as an attractive xeric and pollinator-friendly landscape plant in the Intermountain West (Mee et al. 2003; UW Ext. 2024). Its ready establishment from planted seedlings, rapid growth, showy flowers, and long flowering period make it a landscape favorite (Meyer et al. 2009; UW Ext. 2024). Flowers give off a pleasing scent that, although not strong, resembles a mix between that of orange blossoms and cotton candy (Meyer et al. 2009).
Revegetation Use
Many gooseberryleaf globemallow growth characteristics make it useful in restoration of disturbed or degraded rangelands, mined sites, unstable soils, and for roadside beautification (Wasser 1982). It is a natural colonizer of disturbed sites, tolerates moderate grazing, establishes quickly, exhibits good seedling vigor and competitiveness, fair soil stabilization value, winter hardiness, drought tolerance, and produces seed in year 1 or 2 (Shaw and Monsen 1983; Stevens et al. 1985; Pendery and Rumbaugh 1986; Horton 1989). It is best suited for restoration of sites receiving less than 12 in (305 mm) of annual precipitation (Pendery and Rumbaugh 1993) and has been used successfully in revegetation of pinyon-juniper, sagebrush-rabbitbrush, shadscale, and blackbrush sites (Shaw and Monsen 1983; Stevens et al. 1985; Pendery and Rumbaugh 1986; Horton 1989). Its long flowering period, showy blooms, and low-maintenance growth make it desirable for revegetation of campgrounds, rest areas, visitor centers, and other recreation sites (Shaw and Monsen 1983).
Because of good early growth and rapid reproduction, gooseberryleaf globemallow is often used as a component of wildland seeding mixes (Stevens et al. 1985). Diversity in seed mixes may reduce invasibility of restoration sites. In a field experiment near Spanish Fork, Utah, plots planted with a combination of native shrubs, forbs, and bunchgrasses were invaded less by seeded annual grasses than plots planted with two native bunchgrasses. The experiment used four forb species, one of which was gooseberryleaf globemallow in the more diverse plots. Researchers concluded that invasibility was reduced by structural diversity (Allen and Meyer 2014).
Developing A Seed Supply
For restoration to be successful, the right seed needs to be planted in the right place at the right time. Coordinated planning and cooperation is required among partners to first select appropriate species and seed sources and then properly collect, grow, certify, clean, store, and distribute seed for restoration (PCA 2015).
Developing a seed supply begins with seed collection from native stands. Collection sites are determined by current or projected revegetation requirements and goals. Production of nursery stock requires less seed than large-scale seeding operations, which may require establishment of agricultural seed production fields. Regardless of the size and complexity of any revegetation effort, seed certification is essential for tracking seed origin from collection through use (UCIA 2015).
Seed Sourcing
Because empirical seed zones are also not currently available for gooseberryleaf globemallow, generalized provisional seed zones developed by Bower et al. (2014), may be used to select and deploy seed sources. These provisional seed zones identify areas of climatic similarity with comparable winter minimum temperature and aridity (annual heat:moisture index). In Figure 6, Omernik Level III Ecoregions (Omernik 1987) overlay the provisional seeds zones to identify climatically similar but ecologically different areas. For site-specific disturbance regimes and restoration objectives, seed collection locations within a seed zone and ecoregion may be further limited by elevation, soil type, or other factors.
Barga et al. (2020) conducted an analysis of gooseberryleaf globemallow and other common native forb seeds collected through the national Seeds of Success program. A binary species distribution map developed for gooseberryleaf globemallow indicated a range of 246,248 mi2 (637,783 km2), 8.0% of which had been affected by fire between 2000 and 2018. When climatic conditions (annual precipitation, summer precipitation, and annual maximum and minimum temperatures) at the 20 gooseberryleaf globemallow collection sites were compared with conditions at 500 randomly selected points across the species distribution map, the collection site conditions were similar to the ecological site data. Compared to restoration sites, mean annual precipitation of the collection sites was lower and average maximum temperature greater. Selecting future collection sites with current or predicted climatic conditions comparable to those of areas where restoration activity might be expected was considered critical for meeting future restoration needs in the face of increasing wildfires, weed invasions, and climate change (Barga et al. (2020).
The Western Wildland Environmental Threat Assessment Center’s (USFS WWETAC 2024) Threat and Resource Mapping (TRM) Seed Zone application provides links to interactive mapping features useful for seed collection and deployment planning. The Climate Smart Restoration Tool (Richardson et al. 2020) can also guide restoration planning, seed collection, and seed deployment, particularly when addressing climate change considerations.
Occurrence Map
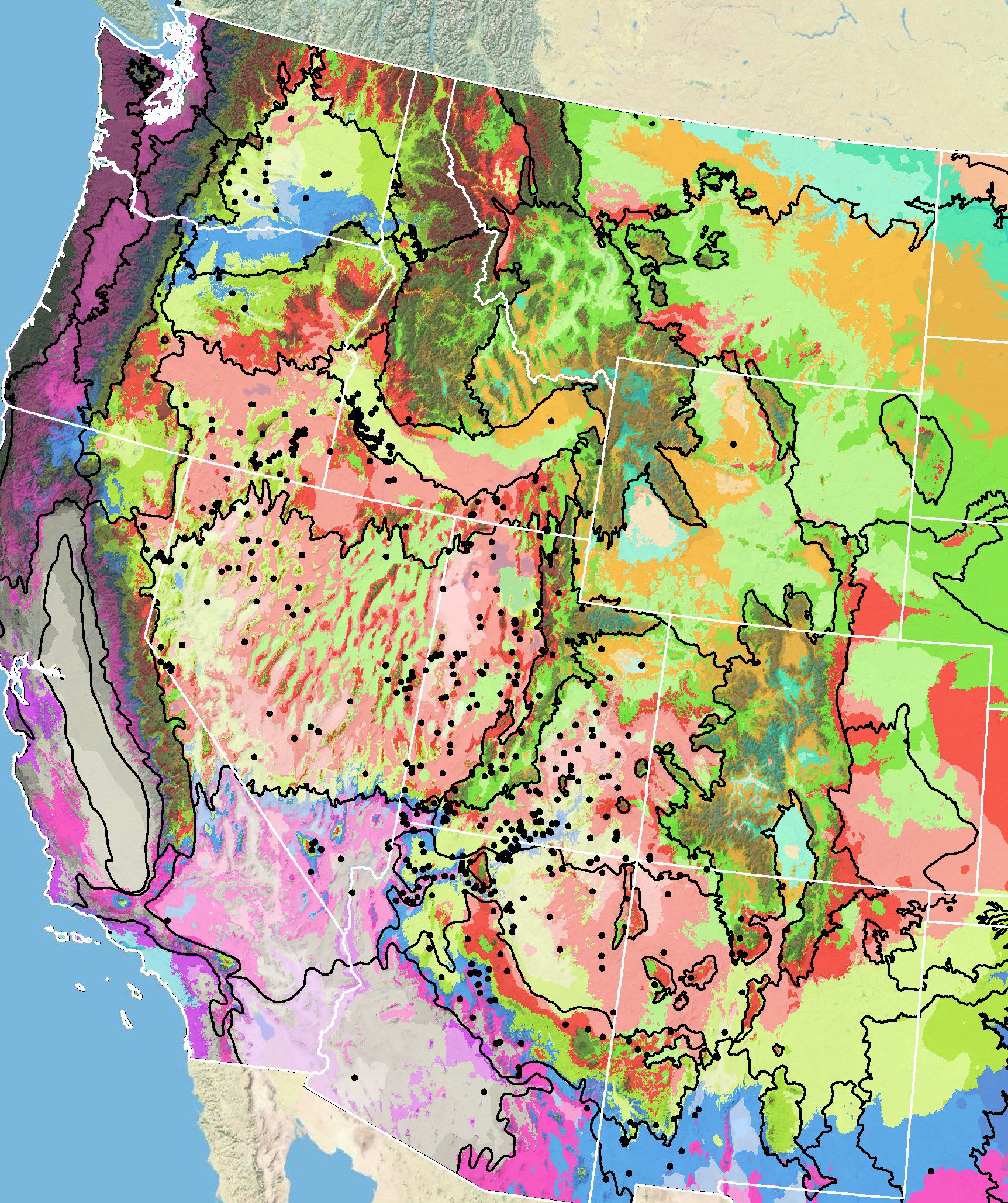
Figure 6. Distribution of gooseberryleaf globemallow (black circles) based on geo-referenced herbarium specimens and observational data from 1902-2016 (CPNWH 2017; SEINet 2017; USGS 2017). Generalized provisional seed zones (colored regions) (Bower et al. 2014) are overlain by Omernik Level III Ecoregions (black outlines) (Omernik 1987; USDI EPA 2018). Interactive maps, legends, and a mobile app are available (USFS WWETAC 2024). Map prepared by M. Fisk, USGS.
Releases
As of early 2024, there were no gooseberryleaf globemallow germplasm releases.
Wildland Seed Collection
Gooseberryleaf globemallow seed ripens unevenly within plants and populations (Shaw and Monsen 1983). Because some seed is dispersed once the fruits are fully mature (Shock et al. 2008), the timing of seed collection is important. Harvests can be maximized by collecting when the lowest schizocarps start to split and the majority are just starting to open. Fruits will be light green-brown in color. At this stage, about 25% of seeds are mature (Pendery and Rumbaugh 1990). Gooseberryleaf globemallow seed can be wildland collected in amounts large enough for local restoration project needs (S. Young, USU retired, personal communication, February 2018). Sometimes seed can be mechanically harvested from wildland stands if stands are large and not especially weedy (Stevens and Monsen 2004b).
Wildland Seed Certification
Verification of species and tracking of geographic source is necessary whether wildland seed is collected for immediate project use or as stock seed for cultivated increase. This official Source Identification process can be accomplished by following procedures established by the Association of Official Seed Certifying Agencies (AOSCA) Pre-Variety Germplasm Program (UCIA 2015; Young et al. 2020). Wildland seed collectors should become acquainted with state certification agency procedures, regulations, and deadlines in the states where they collect.
If wildland-collected seed is to be sold for direct use in ecological restoration projects, collectors must apply for Source-Identified certification prior to making collections. Pre-collection applications, site inspections, and species and seed amount verification are handled by the AOSCA member state agency where seed collections will be made (see listings at AOSCA.org).
If wildland seed collected by a grower or private collector is to be used as stock seed for planting cultivated seed fields or for nursery propagation (See Agricultural Seed Field Certification section), detailed information regarding collection site and collecting procedures must be provided when applying for certification. Photos and herbarium specimens may be required. Germplasm accessions acquired within established protocols of recognized public agencies, however, are normally eligible to enter the certification process as stock seed without routine certification agency site inspections. For contract grow-outs, however, this collection site information must be provided to the grower to enable certification.
Collection Timing
While seeds typically mature in June or August (Plummer et al. 1968; Shaw and Monsen 1983; Stevens et al. 1985; Jorgensen and Stevens 2004), timing of seed maturation can vary widely by location, elevation, plant age, and weather (Wasser 1982; Pendery and Rumbaugh 1990). Wildland seed collections made by Seeds of Success Project field crews ranged from an earliest date of June 7, 2016 at 2,760 ft (840 m) in Owyhee County, Idaho, and a latest date of October 1, 2003 at 6,132 ft (1,869 m) in Kane County, Utah. The most common collection dates fell between mid-June and mid-July (USDI BLM SOS 2017, 2024). In stands established from transplants, flowering was earlier at a northern Utah (early June) than at a southern Idaho site (late June). Flowering date also varied by year and plant age. Average date of first flowering was 1.5 months earlier (mid-July) in the second experiment year (1988) than in the establishment year (1987, early June) in northern Utah and southern Idaho (Pendery and Rumbaugh 1990).
Collection Methods
Seeds can be collected by hand-stripping (Jorgensen and Stevens 2004), which is best in low density or weedy stands. Collectors may also use tennis racquets to swat seed heads over a container to dislodge and collect ripe seed (Fig. 7). For hand-harvesting, gloves are recommended because the hairs covering all parts of the plant can be irritating (USFS GBNPP 2014). Where unusually dense stands occur on nearly level terrain, mechanical harvesting by combine is possible in good production years (Shaw and Monsen 1983).
Several collection guidelines and methods should be followed to maximize the genetic diversity of wildland collections: 1) collect seed from a minimum of 50 randomly selected plants; 2) collect from widely separated individuals throughout a population without favoring the most robust or avoiding small stature plants; and 3) collect from all microsites including habitat edges (Basey et al. 2015). General collecting recommendations and guidelines are provided in online manuals (e.g., ENSCONET 2009; USDI BLM SOS 2023).
It is critical that wildland seed collection does not impact the sustainability of native plant populations. Collectors should take no more than 20% of the viable seed available at the time of harvest (USDI BLM SOS 2024. Additionally, care must be taken to avoid the inadvertent collection of weedy species, particularly those that produce seeds similar in shape and size to those of gooseberryleaf globemallow.
Figure 7. Collecting gooseberryleaf globemallow seed in Sevier County, UT. Photo: S. Young, USU.
Post-Collection Management
Seed should be kept in a dry, shaded place until collections can be moved to a controlled short-term storage environment that is dry, cool, and inaccessible to rodents, weevils (Stevens and Monsen 2004b), or other seed predators. The seed is attractive to weevils (Stevens and Monsen 2004b). If weevils or other insects are suspected in any collection, seed should be frozen for 48 hours or treated with an appropriate insecticide. The more plant material in the collection, the more ventilation and drying a seed lot will likely need (Gold n.d.; Hay and Probert 2011).
Seed Cleaning
Dry seed is easily cleaned (Stevens and Monsen 2004b). Using a gravity table can improve seed purity to over 90% (Stevens et al. 1996).
Several seed cleaning processes have been reported:
- Process dry seed through a debearder, then an air-screen separator, and then a gravity table (Jorgensen and Stevens 2004).
- Process dry seed through a Dybvig seed cleaner and then through a Clipper or Fanning Mill if necessary (Wasser 1982).
- Process dry seed through a brush machine or hammermill and then through an air-screen separator (Tilley et al. 2011).
- Thresh seed using a small-plot combine with an alfalfa seed concave (Shock et al. 2008).
Seed Storage
Seeds are orthodox and can be dried to low moisture contents (SER, INSR, RBG 2024). Seed viability was 95% after dried to a moisture content in equilibrium with 15% relative humidity and stored for 2.2 years at 4 °F (-20 °C) (RBG Kew 2017). Germination of a seed lot stored under ambient conditions in central Utah was low (6–9%) when tested after 2 to 15 years of storage in an open warehouse where temperature extremes ranged from -22 °F (-30 °C) to 100 °F (38 °C). A low germination percentage (2%) of the same seedlot occurred after 25 years of storage. The seed was germinated at 34 to 38 °F (1.1–3.3 °C) in the dark (Stevens and Jorgensen 1994).
Viability Testing
There is an Association of Official Seed Analysts (AOSA) tetrazolium (TZ) viability testing guideline for globemallow species (AOSA 2010) but no germination testing rule (AOSA 2023).
Because some seeds may be hard, they are prepared for treatment by clipping a small piece of the seed coat near the distal end of the cotyledons (AOSA 2010). Clipped seeds are imbibed on moist media overnight at 68 to 77 °F (20-25 °C). Imbibed seeds are prepared for staining by either cutting them deeply, leaving the two halves together and soaking them in 0.1% TZ overnight at 95 °F (35 °C) or by extracting the embryos and soaking them in 1.0% TZ overnight at 95 °F (35 °C). Seeds are counted as viable if the entire embryo and endosperm are evenly stained.
To quickly evaluate seed viability, the ‘pop test’ may be useful. This test uses a hot plate to heat seeds until the moisture contained in the seed is converted to gas and breaks the seed coat. Seeds that pop are those with intact seed coats. Although germination and TZ tests were not done on gooseberryleaf globemallow seeds that popped, the test may still be a good predictor of seed fill (Tilley et al. 2011).
Purity Testing
For routine laboratory analysis, 5 g of seed is required for purity testing and 50 g for noxious weed counts (Belcher 1985).
Germination Biology
Seed is dormant and without pre-treatment low levels of germination can be expected (14-40%) (Young and Young 1986; Whitacre and Call 2006). A variety of physical, mechanical (Page et al. 1966; Roth et al. 1987; Barak et al. 2015), chemical (Page et al. 1966; Roth et al. 1987), and combined treatments (Smith and Kratsch 2009) have been tested to break seed dormancy.
Researchers with experience using gooseberryleaf globemallow in restoration, indicate that erratic germination is common, and maximum germination is not reached until one month after seed is harvested (Stevens et al. 1996). High levels of germination were achieved after nicking seeds and subjecting them to cool moist stratification (87%) (Smith and Kratsch 2009) or a 4-hour soak in diethyl dioxide (67%) (Page et al. 1966), however, these treatments are not practical or feasible on a large scale.
Seeds exhibit double dormancy (Smith and Kratsch 2009). Scarification of the impermeable seed coat is necessary for high initial germination (Tilley et al. 2011). Seeds first need to be made permeable to water through some scarification method (heat or mechanical treatment) followed by stratification to break physiological dormancy (Smith and Kratsch 2009).
Other researchers have also found scarification (acid or mechanical) essential to increase germination (Page et al. 1966). The Royal Botanic Gardens Kew obtained 90 to 95% germination by chipping seed with a scalpel and germinating seeds on 1% agar solutions with alternating light and dark conditions (8/16 hrs) at 68 ºF (20 ºC) and 86 ºF (30 ºC) (SER, INSR, KEW 2024). Boiling water increased seed permeability to water and was better than cool moist stratification alone to break dormancy (Tilley et al. 2011). Germination of seeds after nicking alone was 52%, but when nicked seeds were cool-moist stratified, germination was 87% (Smith and Kratsch 2009). Germination was only 5% with cool moist stratification alone. Acid scarification and 1 to 3 months of cool moist stratification can also be used to germinate seeds (Stevens et al. 1996; Rawlins et al. 2009). Suggested future research includes evaluating other mechanisms and associated timing requirements to break dormancy (Smith and Kratsch 2009).
Wildland Seed Yield And Quality
Post-cleaning seed yield and quality of seed lots collected by Seeds of Success (USDI BLM SOS 2017, 2024) are provided in Table 3 (USFS BSE 2017, 2023). Results indicate that gooseberryleaf globemallow seed can generally be cleaned to high levels of purity and seed fill and that viability of fresh seed is generally high.
Gooseberryleaf globemallow seeds are small, averaging more than 350,000 seeds/lb (770,000 seeds/kg) (Table 4). Other sources (Belcher 1985; Horton 1989; Stevens et al. 1996; Jorgensen and Stevens 2004; USFS GBNPP 2014; Jensen et al. 2022; SER, INSR, KEW 2024) report similar values, ranging from 191,000 to 500,660 seeds/lb (421,000-1,103,750 seeds/kg).
Table 4. Seed yield and quality of gooseberryleaf globemallow seed lots collected in the Intermountain region, cleaned by the Bend Seed Extractory, and tested by the Oregon State Seed Laboratory or the USFS National Seed Laboratory (USFS BSE 2017, 2023).
Characteristic |
Mean |
Range |
Samples (no.) |
Bulk weight (lbs) |
1.1 |
0.14-3.36 |
31 |
Clean weight (lbs) |
0.15 |
0.003-0.61 |
31 |
Clean-out ratio |
0.13 |
0.004-0.30 |
31 |
Purity (%) |
97 |
89-99 |
31 |
Fill (%)¹ |
90 |
45–99 |
31 |
Viability (%)² |
92 |
74-98 |
18 |
Seeds/lb |
297,287 |
146,986-462,850 |
31 |
Pure live seeds/lb |
282,641 |
114,502-378,150 |
18 |
¹100 seed X-ray test
²Tetrazolium chloride test
Marketing Standards
Acceptable seed purity, viability, and germination specifications vary with revegetation plans. Purity needs are highest for precision seeding equipment used in nurseries, while some rangeland seeding equipment handles less clean seed quite well. For gooseberryleaf globemallow seed purchasing, recommended minimum seed viability is 90% and purity is 80% to 90% (Jorgensen and Stevens 2004; Walker and Shaw 2005). As of 2005, the Bureau of Land Management’s minimum requirements for gooseberryleaf globemallow seed purchases were 90% purity, 75% germination, and 0.675 PLS (Lambert 2005). Recommended minimum germination of untreated market seed is 20% (Stevens et al. 1985; Jorgensen and Stevens 2004).
Agricultural Seed Production
In cultivated stands (Fig. 8), plants produce seed within a year of fall planting (Shock et al. 2015), but seed production in the first year may be less than in following years (Stevens et al. 1996). Seed crops can be harvested for 4 to 6 years. Seed is harvested by hand stripping, beating seed into a container, or using a combine harvester (Jorgensen and Stevens 2004). Mowing established plants in the fall concentrates the timing of seed production and provides good yields with a single combine harvest (Shock et al. 2015).
Figure 8. Gooseberryleaf globemallow seed production at Oregon State University’s Malheur Experiment Station in Ontario, OR. Photo: N. Shaw, USFS.
Seed production research plots were grown successfully at the Oregon State University’s Malheur Experiment Station (OSU MES). Plants produced harvestable seed for 5 years (Shock et al. 2012; 2015), but by the sixth year, growth was poor and the plots were eliminated (Shock et al. 2013; 2015). Fall-seeded gooseberryleaf globemallow flowered in the first year. Flowering began in early May and continued through early September (Table 5). Seed was hand-harvested three times (20 June, 10 July, and 13 August) (Shock et al. 2015). When crops were flailed in fall, flowering in the next year was concentrated enough to allow for a single combine harvest (Shock et al. 2015).
Table 5. Flowering dates, harvest dates, and seed yields for seed production fields grown at Oregon State University’s Malheur Experiment Station in Ontario, OR (Shock et al. 2015).
Year |
Start of flowering |
End of flowering |
Harvest date(s) |
Seed yield (lbs/ac) |
2007 |
5 May |
5 Sept |
20 June, 10 July, 13 Aug |
442.3* |
2008 |
5 May |
15 June |
21 July |
275.1 |
2009 |
1 May |
10 June |
14 July |
270.6 |
2010 |
10 May |
25 June |
20 July |
310.4 |
2011 |
26 May |
14 July |
29 July |
223.9 |
*Seed yield represents multiple hand-harvests, which is likely the reason for high yields and not year, plant age, etc.
Agricultural Seed Certification
In order to minimize genetic changes in specific accessions of native species when increased in cultivated fields, it is essential to track the geographic source and prevent inadvertent hybridization or selection pressure. This is accomplished by following third party seed certification protocols for Pre-Variety Germplasm (PVG) as established by the Association of Official Seed Certification Agencies (AOSCA). AOSCA members in the U.S., Canada, and other countries administer PVG requirements and standards that track the source and generation of planting stock. Field and cleaning facility inspections are conducted to monitor stand establishment, proper isolation distances, control of prohibited weeds, seed harvesting, cleaning, sampling, testing, and labeling for commercial sales (UCIA 2015; Young et al. 2020).
Seed growers apply for certification of their production fields prior to planting and plant only certified stock seed of an allowed generation (usually less than four). The systematic and sequential tracking through the certification process requires preplanning, knowing state regulations and deadlines, and is most smoothly navigated by working closely with state certification agency personnel. See the Wildland Seed Certification section for more information on stock seed sourcing.
Site Preparation
Weed-free sites are recommended when planting gooseberryleaf globemallow crops (Stevens et al. 1996).
Seed Pretreatments
If a high level of initial germination is essential, seed requires scarification. There are chemical, mechanical, and physical scarification methods that can be used (see Germination Biology section). When researchers seeded in spring, an overnight soak in a 10% by volume solution of 13% bleach in distilled water followed by about a month of storage at 34 °F (1 °C), failed to mimic fall germination and emergence was poor (Shock et al. 2008).
Weed Management
Weeds in production fields can be controlled by hand, with pre-emergent herbicides, or through cultivation (Stevens et al. 1996). Herbicides are not registered for this species, and the study results presented here do not constitute an endorsement of specific companies or products or recommendations for use. The research, however, could contribute to future registration efforts.
In a controlled study, gooseberryleaf globemallow plants grown in soils treated with an imidazolinone herbicide produced significantly less biomass than those in untreated soils (De Graaff and Johns 2015). When various post-emergence herbicide mixtures were tested on fields in the spring following fall seeding, plant injury ranged from 23% to 95%. A mix of oxyfluorfen and pendimethalin resulted in 23% injury, and all other mixes tested resulted in injury greater than 55%. Researchers concluded plants were very sensitive to herbicides but use of a protective shield to direct spray to target plants, although not tested, may be a management option (Felix et al. 2015).
Seeding
Fall seeding allows for natural stratification and can result in more rapid and uniform germination (Stevens et al. 1996). Spring seeding even with a cold-water soak and cool temperature storage resulted in poor emergence, whereas fall seeding produced good stands at OSU MES (Shock et al. 2008; Shock et al. 2015).
Guidelines for seeding depth range from 0.1 to 0.5 in (0.3–1.3 cm) (Pendery and Rumbaugh 1993; Stevens et al. 1996; Rawlins et al. 2009). Emergence and plant survival may vary with planting depth and soil type (Rawlins et al. 2009). In a greenhouse study, seeding depth had a greater effect on emergence in sandy loam than clay loam field-collected soils. Survival was significantly better in sandy loam (87%) than in clay loam soils (52%), regardless of seeding depth (P = 0.024). Seeding on the soil surface resulted in poor emergence regardless of soil type. Based on emergence and short-term survival (45 days), researchers recommended seeding depths of 0.1 to 0.5 in (0.3-1.3 cm) in clay loams and 0.3 to 0.5 in (0.6-1.3 cm) in sandy loams (Rawlins et al. 2009).
General guidelines for seeding gooseberryleaf globemallow in seed fields include pure stand seeding rates of 20 to 50 PLS/linear ft of row (Stevens et al. 1996; Shock et al. 2008) or 2 lbs PLS/ac (2.3 kg/ha) (Tilley et al. 2011). At a seeding rate of 1 lb PLS/ac (1.1 kg/ha), the average seeding rate is 17 seeds/ft² (183 seeds/m²) (Ogle et al. 2012).
Recommended row spacings range from 28 to 36 in (71-91 cm) (Stevens et al. 1996; Shock et al. 2008). A single-row seeder was used successfully for seed production fields in Utah (Stevens et al. 1996). At OSU MES, good stands were established by drill seeding 30-in (76 cm) rows at 20 to 30 seeds/ft of row. Soils at this site were Nyssa silt loams with a pH of 8.3 and 1.1% organic matter (Shock et al. 2008).
Establishment And Growth
Emerging seedlings produce alternate lobed to rounded leaves. Leaves and stems are grayish-green due to the dense fine hairs (Pavek et al. 2012). The growth, vigor, and survival of seedlings is considered good in field and wildland situations (Stevens and Monsen 2004b; Ogle et al. 2011). Seed is produced in the first year following fall seeding, and seed production fields can be maintained and harvested for up to 6 years, depending on certification requirements (Stevens et al. 1996; Shock et al. 2015). Stand density is rarely a problem, but plant density can fluctuate year to year (Stevens et al. 1996; Stevens and Monsen 2004b). There are thresholds in plant density fluctuations that impact the seed certification process for commercial growers.
Table 6. Seed yield (lbs/ac) for fall-seeded (November 2006) gooseberryleaf globemallow in response to supplemental irrigation. Seed production fields were growing at Oregon State University’s Malheur Experiment Station in Ontario, OR (Shock et al. 2015).
Year | Supplemental irrigation (in/season*) | ||
0 | 4 | 8 | |
Seed yield (lbs/ac) | |||
2007** | 443 | 325 | 352 |
2008 | 276 | 183 | 178 |
2009 | 270 | 299 | 327 |
2010 | 310 | 351 | 346 |
2011 | 224 | 261 | 148 |
*Irrigation season was from bud to seed set.
**Three hand harvests, the following years represent yields from a single combine harvest.
Irrigation
Seed yield for gooseberryleaf globemallow seed production fields at OSU MES were not significantly improved with supplemental irrigation beyond that provided during establishment (Table 6; Shock et al. 2015). Findings suggest moderate levels of seed production occur, regardless of rainfall or supplemental irrigation (Table 7).
Table 7. Natural precipitation (amounts and timing) and growing degree hours at Oregon State University’s Malheur Experiment Station in Ontario, OR (Shock et al. 2012).
Year |
Jan-June (in) |
April-June (in) |
Jan-June hrs 50-86 °F |
2006 |
9.0 |
3.1 |
1120 |
2007 |
3.1 |
1.9 |
1208 |
2008 |
2.9 |
1.2 |
936 |
2009 |
5.8 |
3.9 |
1028 |
2010 |
8.3 |
4.3 |
779 |
2011 |
8.3 |
3.9 |
671 |
Mean |
5.8 |
2.7 |
1042 |
Others have recommended irrigation during establishment and again in the spring and during flowering and seed set at sites receiving 11 to 14 in (279-356 mm) of annual precipitation (Stevens et al. 1996).
Pollinator Management
Gooseberryleaf globemallow is insect-pollinated and especially attractive to bees (Pendery and Rumbaugh 1986; Ogle et al. 2011; Tepedino 2017). Any practice to encourage visits by globemallow bees or other frequent visitors such as Apis, Agapostemon, Calliopsis, Halictus, and Melissodes, and Ammoplanus (Pendery and Rumbaugh 1986; Tepedino 2017) will benefit seed production.
Pest Management
Several microbes, insects, and animals can impact seed production fields. Powdery mildews (Erysiphe plygoni and Leveillula spp.) and rust fungus (Puccinia sherardiana), have been collected from globemallow plants (Sampangi et al. 2010; Mohan and Shock 2014; Farr and Rossman 2023). At OSU MES, rust fungus (P. sherardiana), infected seed production stands over a 2-year period and caused substantial leaf loss and reduced growth (Shock et al. 2011). Weevils (Coleoptera: Apionidae and Cuculionidae) are globemallow seed predators that can severely impact seed production (Pendery and Rumbaugh 1986; CSU Ext. 2016), especially the seed in the indehiscent (lower) part of the fruit (Kearney 1935). When grasshopper (Orthoptera: Caelifera), Mormon cricket (Anabrus simplex), rabbit, and/or rodent population levels are moderate to high, damage to seed production fields, restoration seedings, and established stands can be expected (Wasser 1982).
Seed Harvesting
Seed production fields can be harvested by hand or with mechanical equipment. While multiple hand-harvests will likely result in larger yields than a single mechanical harvest (Shock et al. 2015), a cost-benefit analysis will establish the best method.
In seed production fields in Utah, gooseberryleaf globemallow seed was harvested by handstripping or windrowing then combining (Stevens et al. 1996). Mowing seed production fields in fall encourages more uniform and concentrated flowering, which allows for a single seed harvest using a small-plot combine (Shock et al. 2015). This also helps to ensure harvests from a greater percentage of the plants and serves to increase the genetic diversity of the seed (Basey et al. 2015). Threshing the combine-harvested seed using an alfalfa seed concave produced seed lots that required no additional cleaning (Shock et al. 2008).
Nursery Practice
Nursery production and transplanting of gooseberryleaf globemallow has been successful, and high establishment can be expected from bareroot or container-grown stock (Fig. 9) (Stevens 2004). In greenhouse experiments, Cardoso et al. (2007) found gooseberryleaf globemallow grew well in peat-based media with a pH of 5.5 to 7.2. Negative responses to extremely high or low pH levels were more prevalent in the summer, and plants tolerated lower pH levels (4.5) in the fall and spring, perhaps because of lower temperatures and/or slower growth rates (Cardoso et al. 2007). Plant height and final biomass were not different when plants were grown using conventional above-ground production methods (containers side by side on weed barrier fabric) or a pot-in-pot system (containers placed within larger substrate-filled containers in the ground) (Cardoso et al. 2006).
Average survival was high for gooseberryleaf globemallow (94%) and gooseberryleaf × small-leaf globemallow hybrids (92%) a year after transplanting greenhouse stock (Pendery and Rumbaugh 1990). Stock was planted into clean-tilled sites in Utah and southern Idaho in mid-April. Transplants were grown from sand paper-scarified seed in 9 in³ (150 cm³) cones filled with four parts sand, two parts peat moss, and one part vermiculite. One-year-old transplants weighed an average of 109 g. Weight for all accessions ranged from 64 to 188 g (Pendery and Rumbaugh 1990).
Wildland Seeding And Planting
Gooseberryleaf globemallow is a good candidate for use in wildland revegetation. It tolerates harsh conditions, reproduces early, competes well with cheatgrass and other annuals once established, provides desirable forage, and is attractive to pollinators (Plummer et al. 1968; Stevens et al. 1985; Monsen and Stevens 2004; Eldredge et al. 2013).
While well suited and desirable in wildland restoration, several studies reported poor establishment following seeding. This may be a result of poor establishment conditions that failed to break seed dormancy (see Germination Biology section), use of seed not adapted to the restoration site, or other factors. If rabbit populations are high, establishment of globemallow from seed is challenging (Pendery and Rumbaugh 1986).
Figure 9. Gooseberryleaf globemallow container stock grown by USFS in Provo, UT. Photo: N. Shaw, USFS.
When seeded as part of a mixture on disturbed sites near St. George, Utah, the resulting frequency, density, and cover of gooseberryleaf globemallow were low (Ott et al. 2011). The site was dominated by cheatgrass and prickly Russian thistle (Salsola tragus) before being scalped and drill seeded with native species. Although abundance was low, gooseberryleaf globemallow plants persisted to the last post-treatment visit, which occurred 14 years after seeding. Researchers noted the gooseberryleaf globemallow seed used in the restoration came from commercially available material, which may or may not have been locally adapted (Ott et al. 2011). Establishment was also poor when gooseberryleaf globemallow was used in restoration of a pasture on the Humboldt-Toiyabe National Forest, Nevada. Seed was purchased from a local commercial source and seeded in the fall. Researchers indicated that irrigation, precipitation levels, and their timing may have been insufficient for germination and seedling establishment (Chambers et al. 2014).
The effects of seeding treatments and environmental factors were examined for 20 native forb species in two years (2013 and 2014) at three sites (Wells and Orovada, Nevada, and Fountain Green, Utah) (Jensen et al. 2022). Treatments combinations were seeding depth (1.4, 2.6, 3.6 or 4 cm) and row cover (present or absent). Row cover increased emergence by more than 99% at Fountain Green in 2013, and by 11% in 2014. Emergence of planted seed at Orovada was inadequate to quantify for all plots in 2013 and for plots lacking row cover in 2014. Emergence at Orovada with row cover was 1 ± 0.75% in 2014. Emergence was too low to evaluate at Wells in either year (Jensen et al. 2022). Row cover was not considered practical for large-scale seedings but could be used for establishing forb islands.
Seeding depth increases had a negative effect on emergence at Fountain Green in 2013 (-24.9 %) (Jensen 2022). In 2014 seeding depth increases had a positive effect at Fountain Green (+110.3%) and at Orovada (+40.7%). Increases in emergence at greater depths in 2014 were possibly related to lower precipitation at both sites during the March 1 to April 21 emergence period in 2014 compared to the same period in 2013.
Landeen et al. (2021) examined the potential for creating self-sustaining islands of native forbs using N-sulate ground cover fabric to increase soil moisture and temperature to improve seedbed conditions. Plots (5 ft x 25 ft [1.5 m x 7.6 m], 5 replications per site) were established at three Wyoming big sagebrush and one mountain big sagebrush site in central and south-central Utah. Elevation of the sites ranged from 5,300 to 7,200 ft (1,613-2,187 m) and precipitation ranged from 13.0 to 14.1 in (331-359 mm). Gooseberryleaf globemallow was seeded at a rate of 4.29 PLS/ft2 (46.18 PLS/m2) in a mix with six other native forbs and one native grass (total seeding rate 31.57 PLS/ft2 [339.8 PLS/ m2]). Plant density was evaluated by species in post-seeding years 1, 2, and 5. Across all sites, gooseberryleaf globemallow emergence in year 1 was improved with ground cover (0.21 plants/m2 with cover vs 0.04 plants/m2 without, p = 0.0380). Plant density increased in plots with and without ground cover in years 2 and 5, but no treatment difference was found in either year (year 2: mean =0.08 plants/m2, p = 0.9766; year 5: mean = 0.46 plants/m2, p = 0.2279) (Landeen et al. (2021).
Seeding and Planting Methods
Wildland seeding recommendations for Nevada suggest use on sites where soil pH ranges from 5 to 7 and there is no salinity (Eldredge et al. 2013). However, in native habitats, plants occur on moderately saline soils (Wasser 1982), and plants are frequently found on basic soils (Kearney 1935; Horton 1989; Pendery and Rumbaugh 1993).
Seeding in the fall or winter provides the best opportunity to naturally break seed dormancy (Shaw and Monsen 1983). Broadcast, drill, surface compacting, or browse interseeding are all workable seeding methods (Monsen and Stevens 2004), but seed coverage improves emergence (Stevens et al. 1985; Rawlins et al. 2009). Although recommended for seeding in mixtures and often described as competitive, gooseberryleaf globemallow may not do as well when seeded with aggressive non-native cultivars. Although gooseberryleaf globemallow establishment was good when seeded in April with ‘Hycrest’ crested wheatgrass in northern Utah and southern Idaho, its density dropped dramatically in the years following the establishment year (Pendery and Rumbaugh 1990).
In seed mixes, where gooseberryleaf globemallow may comprise 10% or less of the mixture (Wasser 1982), recommended seeding rates range from 0.75 to 3 lbs/ac (0.64-2.6 kg/ha) (Kitchen 1994). Increasing the seeding rate has not been successful in increasing establishment and survival (Monsen and Stevens 2004). Recommended seeding rates, however, vary by the potential climax vegetation of a site and the seeding method (Plummer et al. 1968). Rates are typically lower for drill seeding than for broadcast seeding methods. In Utah, seeding has been successful on pinyon-juniper, sagebrush-rabbitbrush, shadscale, and blackbrush sites and in disturbed areas with exposed, eroded soils and harsh environments. The recommended seeding rate for use in blackbrush or shadscale/winterfat (Krascheninnikovia lanata) vegetation is 1 lb PLS/ac (0.9 kg/ha) and in fourwing saltbush (Atriplex canescens)/big sagebrush communities with spiny hopsage (Grayia spinosa) is 2 lbs PLS/ac (2.3 kg/ha) (Horton 1989). On shadscale sites, 1.5 lbs PLS/ac (1.3 kg/ha) is recommended for broadcast seeding and 1 lb PLS/ac for drill seeding. On blackbrush sites, 1 lb PLS/ac (0.9 kg/ha) is recommended for broadcast seeding and 0.5 lb/ac (0.4 kg/ha) for drill seeding (Plummer et al. 1968). For drill seeding, the recommended seeding depth is 0.1 to 0.3 in (0.3-0.8 cm) and row spacing is 15 to 18 in (38-46 cm) (Monsen and Stevens 2004). However, adjustments based on soil type may improve emergence. Seeded areas should not be grazed for at least two growing seasons after seeding (Stevens et al. 1985). Seedlings can be damaged by heavy grazing.
Acknowledgements
Funding for Western Forbs: Biology, Ecology, and Use in Restoration was provided by the USDI BLM Great Basin Native Plant Materials Ecoregional Program through the Great Basin Fire Science Exchange. Great thanks to the chapter reviewers: Stan Young, Utah State University, and Jan Gurr, USFS (retired).
This research was supported in part by the USDA Forest Service, Rocky Mountain Research Station. The findings and conclusions in this publication are those of the authors and should not be construed to represent any official USDA or U.S. Government determination or policy.
Literature Cited
Allen, P.S.; Meyer, S.E. 2014. Community structure affects annual grass weed invasion during restoration of a shrub-steppe ecosystem. Invasive Plant Science and Management. 7(1): 1-13.
Alzerreca-Angelo, H.; Schupp, E.W.; Kitchen, S.G. 1998. Sheep grazing and plant cover dynamics of a shadscale community. Journal of Range Management. 51(2): 214-222.
Association of Official Seed Analysts [AOSA]. 2010. AOSA/SCST Tetrazolium testing handbook. Contribution No. 29. Lincoln, NE: Association of Official Seed Analysts.
Association of Official Seed Analysts [AOSA]. 2023. AOSA rules for testing seeds. Vol. 1. Principles and procedures. Washington, DC: Association of Seed Analysts.
Atwood, N.D.; Welsh, S.L. 2002. Overview of Sphaeralcea (Malvaceae) in southern Utah and northern Arizona, U.S.A., and description of a new species. Novon. 12(2): 159-166.
Barak, R.S.; Fant, J.B.; Kramer, A.T.; Skogen, K.A. 2015. Assessing the value of potential “native winners” for restoration of cheatgrass-invaded habitat. Western North American Naturalist. 75(1): 58-69.
Basey, A.C.; Fant, J.B.; Kramer, A.T. 2015. Producing native plant materials for restoration: 10 rules to collect and maintain genetic diversity. Native Plants Journal. 16(1): 37-53.
Beale, D.M.; Smith, A.D. 1970. Forage use, water consumption, and productivity of pronghorn antelope in western Utah. The Journal of Wildlife Management. 34(3): 570-582.
Belcher, E. 1985. Handbook on seeds of browse-shrubs and forbs. Tech. Pub. No. R8-TP8. Atlanta, GA: U.S. Department of Agriculture, Forest Service, Southern Region. 246 p.
Blackwell, L.R. 2006. Great Basin wildflowers: A guide to common wildflowers of the high deserts of Nevada, Utah, and Oregon. Helena, MT: Morris Book Publishing. 288 p.
Blaisdell, J.P.; Holmgren, R.C. 1984. Managing Intermountain rangelands – Salt-desert shrub ranges. Gen. Tech. Rep. INT-163. Ogden, UT: U.S. Department of Agriculture, Forest Service, Intermountain Forest and Range Experiment Station. 56 p.
Bower, A.D.; St. Clair, J.B.; Erickson, V. 2014. Generalized provisional seed zones for native plants. Ecological Applications. 24(5): 913-919.
Callison, J.; Brotherson, J.D.; Bowns, J.E. 1985. The effects of fire on the blackbrush (Coleogyne ramosissima) community of southwestern Utah. Journal of Range Management. 38(6): 535-538.
Cane, J.H.; Love, B. 2016. Floral guilds of bees in sagebrush steppe: Comparing bee usage of wildflowers available for postfire restoration. Natural Areas Journal. 36(4): 377-391.
Cardoso, G.; Cerny-Koenig, T.; Koenig, R.; Kjelgren, R. 2007. Characterizing fertilizer and media pH requirements for greenhouse production of Intermountain West native herbaceous perennials. Native Plants Journal. 8(2): 115-121.
Cardoso, G.; Kjelgren, R.; Cerny-Koenig, T.; Koenig, R. 2006. Pot-in-pot production of six Intermountain West native herbaceous perennial species grown in containers. Journal of Environmental Horticulture. 24(2): 77-83.
Chambers, J.C.; Eldredge, E.P.; Snyder, K.A.; Board, D.I.; Forbis de Queiroz, T.; Hubbard, V. 2014. Restoring abandoned agricultural lands in cold desert shrublands: tradeoffs between water availability and invasive species. Invasive Plant Science and Management. 7(1): 176-189.
Colorado State University Extension [CSU Ext.]. 2016. Western Colorado insects: Native plant seed production. http://www.wci.colostate.edu/seed_production.shtml
Consortium of Pacific Northwest Herbaria [CPNWH]. 2017. Seattle, WA: University of Washington Herbarium, Burke Museum of Natural History and Culture. http://www.pnwherbaria.org/index.php
De Graaff, M.; Johns, A. 2015. Herbicide impacts on forb performance in degraded sagebrush steppe ecosystems. In: Kilkenny, F; Halford, A.; Malcomb, A., eds. Great Basin Native Plant Project: 2014 Progress Report. Boise, ID: U.S. Department of Agriculture, Forest Service, Rocky Mountain Research Station: 60-68.
Donart, G.B. 1994. SRM 501: Saltbush-greasewood. In: Shiflet, T.D., ed. Rangeland cover types of the United States. Denver, CO: Society for Range Management: 61-62.
Dumroese, R.K.; Luna, T.; Pinto, J.R.; Landis, T.D. 2016. Forbs: Foundation for restoration of monarch butterflies, other pollinators, and greater sage-grouse in the western United States. Native Plants Journal. 36(4): 499-511.
Eldredge, E.; Novak-Echenique, P.; Heater, T.; Mulder, A.; Jasmine, J. 2013. Plants for pollinator habitat in Nevada. Tech. Note NV 57. Reno, NV: U.S. Department of Agriculture, Natural Resources Conservation Service. 65 p.
European Native Seed Conservation Network [ENSCONET]. 2009. ENSCONET seed collecting manual for wild species. Edition 1: 32 p.
Farr, D.F.; Rossman, A.Y. 2023. Fungal databases, U.S. National Fungus Collections. U.S. Department of Agriculture, Agricultural Research Service. https://fungi.ars.usda.gov/
Fautin, R.W. 1946. Biotic communities of the northern desert shrub biome in western Utah. Ecological Monographs. 16(4): 251-310.
Felix, J.; Ishida, J.; Feibert, E.; Rivera, A. 2015. Forb response to post-emergence herbicides. In: Kilkenny, F; Halford, A.; Malcomb, A., eds. Great Basin Native Plant Project: 2014 Progress Report. Boise, ID: U.S. Department of Agriculture, Forest Service, Rocky Mountain Research Station: 101-103.
Flowers, S. 1934. Vegetation of the Great Salt Lake region. Botanical Gazette. 95(3): 353-418.
Gold, K. n.d. Post-harvest handling of seed collections. Tech. Information Sheet 04. UK: Royal Botanic Gardens Kew and Millennium Seed Bank Partnership. 4 p.
Goodrich, S.; Neese, E. 1986. Uinta Basin flora. Ogden, UT: U.S. Department of Agriculture, Forest Service, Intermountain Region. 320 p.
Hancock, Norman V. 1966. Wildlife use of the salt desert shrub areas of the Great Basin. In: Salt desert shrub symposium: Proceedings; 1966 August 1-4; Cedar City, UT. Washington, DC: U.S. Department of the Interior, Bureau of Land Management: 101-112.
Hay F.R.; Probert, R.J. 2011. Chapter 20: Collecting and handling seeds in the field. In: Guarino, L.; Ramanatha, V.; Goldberg, E. Collecting plant genetic diversity: Technical Guidelines-2011 update. Rome, Italy: Bioversity International. 33 p.
Hitchcock, C.L.; Cronquist, A. 2018. Flora of the Pacific Northwest: An illustrated manual. Second Ed. Giblin, D.E.; Legler, B.S.; Zika, P.F.; Olmstead, R.G., eds. Seattle, WA: University of Washington Press. 882 p.
Holmgren, N.H.; Holmgren, P.K.; Cronquist, A. 2005. Intermountain flora: Vascular plants of the Intermountain West, U.S.A. Volume Two, Part B: Subclass Dilleniidae. Bronx, NY: New York Botanical Garden Press. 488 p.
Horton, H. 1989. Interagency forage and conservation planting guide for Utah. AG-433. Logan, UT: Utah State University Extension. 84 p.
Hutchings, S.S.; Stewart, G. 1953. Increasing forage yields and sheep production on Intermountain winter ranges. Circular 925. Washington, D.C.: U.S. Department of Agriculture, Forest Service. 63 p.
ITIS Database. 2024. Integrated Taxonomic Information System. Available: http://www.itis.gov/index.html
Jensen, S.; Christensen, W.F.; Roundy, B.; Anderson, V.J.; Kitchen, S.G.; Allphin, L. 2022. Temporal and spatial factors influence native forb emergence more than sowing depth. Rangeland Ecology and Management. 83: 41-49.
Jorgensen, K.R.; Stevens, R. 2004. Seed collection, cleaning, and storage. In: Monsen, S.B.; Stevens, R.; Shaw, N.L., comps. Restoring western ranges and wildlands, vol. 3. Gen. Tech. Rep. RMRS-GTR-136-vol-3. Fort Collins, CO: U.S. Department of Agriculture, Forest Service, Rocky Mountain Research Station: 699-716.
Kearney, T.H. 1935. The North American species of Sphaeralcea subgenus Eusphaeralcea. Berkeley, CA: University of California Press. 127 p.
Kitchen, S.G. 1994. Perennial forb life-history strategies on semiarid rangelands: Implications for revegetation. In: Monsen, S.B.; Kitchen, S.G., comps. Proceedings – Ecology and management of annual rangelands; 1992 May 18-22; Boise, ID. Gen. Tech. Rep INT-GTR-313. Ogden, UT: U.S. Department of Agriculture, Forest Service, Intermountain Research Station: 342-346.
Kitchen, S.G.; Hall, D.B. 1996. Community stability in a salt-desert shrubland grazed by sheep: The Desert Experimental Range story. In: Barrow, J.R.; McArthur, E.D.; Sosebee, R.E.; Tausch, R.J., comps. Proceedings: Shrubland ecosystem dynamics in a changing environment; 1995 May 23-25; Las Cruces, NM. INT-GTR-338. Ogden, UT: U.S. Department of Agriculture, Forest Service, Intermountain Research Station: 102-107.
Kufeld, R.C. 1973. Foods eaten by the Rocky Mountain elk. Journal of Range Management. 26(2): 106-113.
Kufeld, R.C.; Wallmo, O.C.; Feddema, C. 1973. Foods of the Rocky Mountain mule deer. Res. Pap. RM-111. Fort Collins, CO: U.S. Department of Agriculture, Forest Service, Rocky Mountain Forest and Range Experiment Station. 31 p.
La Duke, J. 2016. 48. Sphaeralcea. In: Flora of North America Editorial Committee, ed. Flora of North America North of Mexico. Volume 6 Malvaceae. http://www.efloras.org
Lady Bird Johnson Wildflower Center [LBJWC]. 2023. Sphaeralcea grossulariifolia (Hook. & Arn.) Rydb. Native Plant Database. Austin, TX: Lady Bird Johnson Wildflower Center. https://www.wildflower.org/plants [Accessed 2024 May 2].
Lambert, S. 2005. Guidebook to the seeds of native and non-native grasses, forbs and shrubs of the Great Basin. Boise, ID: U.S. Department of the Interior, Bureau of Land Management, Idaho State Office. 136 p.
Landeen, M.; Jones, C.; Jensen, S.; Whittaker, A.; Summers, D.D.; Eggett, D.; Petersen, S.L. 2021. Establishing seed islands for native forb species on rangelands using N-Sulate ground cover fabric. Native Plants Journal. 22(1): 51-63.
Love, B.; Cane, J. 2019. Mortality and flowering of Great Basin perennial forbs after experimental burning: Implications for wild bees. Rangeland Ecology and Management. 72(2): 310-317.
Mee, W.; Barnes, J.; Kjelgren, R.; Sutton, R.; Cerny, T.; Johnson, C. 2003. Water wise: Native plants for Intermountain landscapes. Logan, UT: Utah State University Press. 220 p.
Meyer, S.E.; Kjelgren, R.K.; Morrison, D.G.; Varga, W.A. 2009. Chapter 7: The plant palette. In: Landscaping on the new frontier: waterwise design for the Intermountain West. Logan, UT: Utah State University Press. 159-226.
Moerman, D. 2003. Native American ethnobotany: A database of foods, drugs, dyes, and fibers of Native American peoples, derived from plants. Dearborn, MI: University of Michigan. http://naeb.brit.org
Mohan, S.K.; Shock, C.C. 2014. Etiology, epidemiology, and management of diseases of native wildflower seed production. In: Kilkenny, F.; Shaw, N.L.; Gucker, C.L., eds. Great Basin Native Plant Project: 2013 Progress Report. Boise, ID: U.S. Department of Agriculture, Forest Service, Rocky Mountain Research Station: 107-108.
Monsen, S.B.; Stevens, R. 2004. Seedbed preparation and seeding practices. In: Monsen, S.B.; Stevens, R.; Shaw, N.L., comps. Restoring western ranges and wildlands, vol. 1. Gen. Tech. Rep. RMRS-GTR-136-vol-1. Fort Collins, CO: U.S. Department of Agriculture, Forest Service, Rocky Mountain Research Station: 121-154.
Morris, L.R.; Monaco, T.A.; Leger, E.; Blank, R.; Sheley, R. 2013. Cultivation legacies alter soil nutrients and differentially affect plant species performance nearly a century after abandonment. Plant Ecology. 214(6): 831-844.
Ogle, D.; St. John, L.; Stannard, M.; Holzworth, L. 2012. Conservation plant species for the Intermountain West. Plant Materials Technical Note 24. Boise, ID: U.S. Department of Agriculture, Natural Resources Conservation Service. 57 p.
Ogle, D.; Tilley, D.; Cane, J.; St. John, L.; Fullen, K.; Stannard, M.; Pavek, P. 2011. Plants for pollinators in the Intermountain West. Plant Materials Tech. Note 2A. Boise, ID: U.S. Department of Agriculture, Natural Resources Conservation Service. 40 p.
Omernik, J.M. 1987. Ecoregions of the conterminous United States. Map (scale 1:7,500,000). Annals of the Association of American Geographers. 77(1): 118-125.
Ott, J.; McArthur; E.D.; Roundy, B. 2003. Vegetation of chained and non-chained seedings after wildfire in Utah. Journal of Range Management. 56: 81-91.
Ott, J.E.; McArthur, E.D.; Sanderson, S.C. 2001. Plant community dynamics of burned and unburned sagebrush and pinyon-juniper vegetation in west-central Utah. In: McArthur, E. D.; Fairbanks, D. J., comps. Shrubland ecosystem genetics and biodiversity: Proceedings; 2000 June 13-15; Provo, UT. Proc. RMRS-P-21. Ogden, UT: U.S. Department of Agriculture, Forest Service, Rocky Mountain Research Station: 177-191.
Ott, J.E.; McArthur, E.D.; Sanderson, S.C. 2011. Vegetation dynamics at a Mojave Desert restoration site, 1992 to 2007. Natural Resources and Environmental Issues. 16(15): 1-16.
Page, R.J.; Goodwin, D.L.; West, N.E. 1966. Germination requirements of scarlet globemallow. Journal of Range Management. 19(3): 145-146.
Parkinson, H. 2003. Landscaping with native plants of the Intermountain Region. Tech. Ref. 1730-3. Boise, ID: U.S. Department of the Interior, Bureau of Land Management. 46 p.
Pase, C.P.; Knipe, O.D. 1977. Effect of winter burning on herbaceous cover on a converted chaparral watershed. Journal of Range Management. 30(5): 346-348.
Pavek, P.; Erhardt, B.; Heekin, T.; Old, R. 2012. Forb seedling identification guide for the Inland Northwest: Native, introduced, invasive, and noxious species. Pullman, WA: U.S. Department of Agriculture, Natural Resources Conservation Service, Pullman Plant Materials Center. 144 p.
Pendery, B.M.; Rumbaugh, M.D. 1986. Globemallows: Forbs for Utah rangelands. Utah Science: 41-45.
Pendery, B.M.; Rumbaugh, M.D. 1990. Survival and growth of globemallow (Sphaeralcea) species in dry land spaced-plant nurseries. Journal of Range Management. 43(5): 428-432.
Pendery, B.M.; Rumbaugh, M.D. 1993. Globemallows. Rangelands. 15(3): 127-130.
Plant Conservation Alliance [PCA]. 2015. National seed strategy for rehabilitation and restoration 2015-2020. Washington, DC: U.S. Department of the Interior, Bureau of Land Management. 52 p.
Plummer, A.P. 1958. Restoration of juniper-pinyon ranges in Utah. In: Meyer, A.B.; Eyre, F.H., eds. Proceedings, Society of American Foresters Meeting: Multiple-use forestry in the changing West; 1958 September 28-October 2; Salt Lake City, UT. Washington, D.C.: Society of American Foresters: 207-211.
Plummer, A.P.; Christensen, D.R.; Monsen, S.B. 1968. Restoring big-game range in Utah. Pub. 68-3. Salt Lake City, UT: Utah Division of Fish and Game. 183 p.
Rawlins, J.K.; Anderson, V.J.; Johnson, R.; Krebs, T. 2009. Optimal seeding depth of five forb species from the Great Basin. Native Plants Journal. 10(1): 33-42.
Richardson, B.; Kilkenny, F.; St. Clair, B.; Stevenson-Molnar, N. 2020. Climate Smart Restoration Tool. https://climaterestorationtool.org/csrt/
Roth, T.E.; Holechek, J.L.; Hussain, M.Y. 1987. Germination response of three globemallow species to chemical treatment. Journal of Range Management. 40(2): 173-175.
Rumbaugh, M.D.; Mayland, H.F.; Pendery, B.M.; Shewmaker, G.E. 1993a. Element concentrations in globemallow herbage. Journal of Range Management. 46(2): 114-117.
Rumbaugh, M.D.; Mayland, H.F.; Pendery, B.M.; Shewmaker, G.E. 1993b. Utilization of globemallow (Sphaeralcea) taxa by sheep. Journal of Range Management 46(2): 103-109.
Sampangi, R.; Aime, M.C.; Mohan, K.; Shock, C. 2010. New and re-emerging rust diseases from Idaho and Oregon. Phytopathology. 100(S113).
SEINet – Regional Networks of North American Herbaria Steering Committee [SEINet]. 2017. SEINet Regional Networks of North American Herbaria. https://symbiota.org/seinet/
Sharp, L.A.; Sanders, K.; Rimbey, N. 1990. Forty years of change in a shadscale stand in Idaho. Rangelands. 12(6): 313-328.
Shaw, N.; Monsen, S.B. 1983. Nonleguminous forbs for rangeland sites. In: Monsen, S.B; Shaw, N. Managing Intermountain rangelands-Improvement of range and wildlife habitats; 1981 September 15-17; Twin Falls, ID; 1982 June 22-24; Elko, NV. Gen. Tech. Rep. INT-157. Ogden, UT: U.S. Department of Agriculture, Forest Service, Intermountain Forest and Range Experiment Station: 123-131.
Shock, C.; Feibert, E.; Saunders, L.; Shaw, N. 2008. Subsurface drip irrigation for native wildflower seed production. In: Shock, C., ed. Malheur Experiment Station Annual Report 2007. Special Report 1087. Corvallis, OR: Oregon State University: 183-196.
Shock, C.; Feibert, E.; Saunders, L.; Shaw, N.; Sampangi, R.S. 2012. Irrigation requirements for native wildflower seed production. In: Shock, C., ed. Malheur Experiment Station Annual Report 2011. OSU AES Ext/CrS 141. Corvallis, OR: Oregon State University: 139-160.
Shock, C.; Feibert, E.; Shaw, N.; Sampangi, R.S. 2011. Native wildflower seed production with low levels of irrigation. In: Shock, C., ed. Malheur Experiment Station Annual Report 2010. OSU AES Ext/CrS 132. Corvallis, OR: Oregon State University: 158-178.
Shock, C.C.; Feibert, E.B.G.; Saunders, L.D.; Shaw, N.; Sampangi, R.S. 2013. Eight years evaluating the irrigation requirements for native wildflower seed production. In: Shock, C.C, ed. Malheur Experiment Station Annual Report 2012. OSU AES Ext/CrS 144. Corvallis, OR: Oregon State University: 138-159.
Shock, C.C.; Feibert, E.B.G.; Shaw, N.L.; Shock, M.P.; Saunders, L.D. 2015. Irrigation to enhance native seed production for Great Basin restoration. Natural Areas Journal. 35(1): 74-82.
Shreve, F.; Hinckley, A.L. 1937. Thirty years of change in desert vegetation. Ecology. 18(4): 463-478.
Smith, A.; Kratsch, H. 2009. Seed propagation of Sphaeralcea (globemallow). HortScience. 44(4): 1139-1140.
Society for Ecological Restoration; International Network for Seed Based Restoration; Royal Botanic Gardens [SER, INSR, RBGK]. 2024. Seed Information Database (SID). Available from: https://ser-sid.org/
Sriladda, C.; Kratsch, H.A.; Larson, S.R.; Kjelgren, R.K. 2012. Morphological and genetic variation among four high desert Sphaeralcea species. Hortscience. 47(6): 715-720.
Stevens, R. 2004. Establishing plants by transplanting and interseeding. In: Monsen, S.B.; Stevens, R.; Shaw, N.L., comps. Restoring western ranges and wildlands, vol. 3. Gen. Tech. Rep. RMRS-GTR-136-vol-3. Fort Collins, CO: U.S. Department of Agriculture, Forest Service, Rocky Mountain Research Station: 739-744.
Stevens, R.; Jorgensen, K.R. 1994. Rangeland species germination through 25 and up to 40 years of warehouse storage. In: Monsen, S.B.; Kitchen, S.G., comps. Proceedings- Ecology and management of annual rangelands; 1992 May 18-22; Boise, ID. Gen. Tech. Rep INT-GTR-313. Ogden, UT: U.S. Department of Agriculture, Forest Service, Intermountain Research Station: 257-265.
Stevens, R.; Jorgensen, K.R.; Young, S.A.; Monsen, S.B. 1996. Forb and shrub seed production guide for Utah. 1996. AG 501. Logan, UT: Utah State University Extension. 52 p.
Stevens, R.; Monsen, S.B. 2004a. Forbs for seeding range and wildlife habitats. In: Monsen, S.B.; Stevens, R.; Shaw, N.L., comps. Restoring western ranges and wildlands, vol. 2. Gen. Tech. Rep. RMRS-GTR-136-vol-2. Fort Collins, CO: U.S. Department of Agriculture, Forest Service, Rocky Mountain Research Station: 425-466.
Stevens, R.; Monsen, S.B. 2004b. Guidelines for restoration and rehabilitation of principal plant communities. In: Monsen, S.B.; Stevens, R.; Shaw, N.L., comps. Restoring western ranges and wildlands, vol. 1. Gen. Tech. Rep. RMRS-GTR-136-vol-1. Fort Collins, CO: U.S. Department of Agriculture, Forest Service, Rocky Mountain Research Station: 199-294.
Stevens, R.; Shaw, N.; Howard, C.G. 1985. Important nonleguminous forbs for Intermountain ranges. In: Proceedings: Selected papers presented at the 38th Annual Meeting of the Society for Range Management; 1985 February 11-15; Salt Lake City, UT. Denver, CO: Society for Range Management: 102-112.
Tepedino, V. 2017. Globe mallow bee (Diadasia diminuta). Washington, DC: U.S. Department of Agriculture, Forest Service, Rangeland Management and Vegetation Ecology-Botany Program. https://www.fs.fed.us/wildflowers/pollinators/pollinator-of-the-month/globe_mallow_bee.shtml2017 [Accessed 2017 October 13].
Tilley, D.; St. John, L.; Ogle, D.; Shaw, N. 2011. Plant guide: Gooseberryleaf globemallow (Sphaeralcea grossulariifolia [Hook. & Arn.] Rydb.). Aberdeen, ID: U.S. Department of Agriculture, Natural Resources Consrvation Service, Aberdeen Plant Materials Center. 4 p.
University of Wyoming Extension [UW Ext.] 2024. Native plants for the Intermountain West. Laramie, WY: University of Wyoming. https://cwelwnp.usu.edu/westernnativeplants/
USDA Forest Service, Bend Seed Extractory [USDA FS BSE]. 2017. Nursery Management Information System Version 4.1.11. Local Source Report 34-Source Received. Bend, OR: U.S. Department of Agriculture, Forest Service, Bend Seed Extractory.
USDA Forest Service, Bend Seed Extractory [USDA FS BSE]. 2023. Nursery Management Information System Version 4.1.11. Local Source Report 34-Source Received. Bend, OR: U.S. Department of Agriculture, Forest Service Bend Seed Extractory.
USDA Forest Service, Great Basin Native Plant Project [USFS GBNPP]. 2014. Seed weight table calculations made in-house. Report on file. Boise, ID: U.S. Department of Agriculture, Forest Service, Boise Aquatic Sciences Laboratory. Available: https://www.fs.fed.us/rm/boise/research/shrub/Links/Seedweights.pdf
USDA Forest Service, Western Wildland Environmental Threat Assessment Center [USFS WWETAC]. 2017. TRM Seed Zone Applications. Prineville, OR: U.S. Department of Agriculture, Forest Service, Western Wildland Environmental Threat Assessment Center. https://www.fs.fed.us/wwetac/threat-map/TRMSeedZoneMapper.php
USDA Forest Service, Western Wildland Environmental Threat Assessment Center [USFS WWETAC]. 2024. TRM Seed Zone Applications. Prineville, OR: U.S. Department of Agriculture, Forest Service, Western Wildland Environmental Threat Assessment Center. Available: https://www.fs.usda.gov/wwetac/threat-map/TRMSeedZoneMapper.php
USDA Natural Resources Conservation Service [USDA NRCS]. 2024. The PLANTS Database. Greensboro, NC: U.S. Department of Agriculture, Natural Resources Conservation Service, National Plant Data Team. https://plants.usda.gov/home
USDI Bureau of Land Management, Seeds of Success [USDI BLM SOS]. 2017. Seeds of Success collection data. Washington, DC: U.S. Department of the Interior, Bureau of Land Management, Plant Conservation and Restoration Program.
USDI Bureau of Land Management, Seeds of Success [USDI BLM SOS]. 2023. Bureau of Land Management technical protocol for the collection, study, and conservation of seeds from native plant species for Seeds of Success. Washington, DC: U.S. Department of the Interior, Bureau of Land Management, Plant Conservation and Restoration Program. 45 p.
USDI Bureau of Land Management, Seeds of Success [USDI BLM SOS]. 2024. Seeds of Success collection data. Washington, DC: U.S. Department of the Interior, Bureau of Land Management, Plant Conservation and Restoration Program.
USDI Environmental Protection Agency [USDI EPA]. 2017. Ecoregions. Washington, DC: U.S. Department of the Interior, Environmental Protection Agency. https://www.epa.gov/eco-research/ecoregions
USDI Geological Survey [USDI USGS]. 2017. Biodiversity Information Serving Our Nation (BISON). U.S. Geological Survey. https://bison.usgs.gov/#home
Utah Crop Improvement Association [UCIA]. 2015. How to be a seed connoisseur. Logan, UT: UCIA, Utah Department of Agriculture and Food, Utah State University and Utah State Seed Laboratory. 16 p.
Walker, S.C.; Shaw, N.L. 2005. Current and potential use of broadleaf herbs for reestablishing native communities. In: Shaw, N.L.; Pellant, M.; Monsen, S.B., comps. Sage-grouse habitat restoration symposium proceedings; 2001 June 4-7; Boise, ID. RMRS-P-38. Fort Collins, CO: U.S. Department of Agriculture, Forest Service, Rocky Mountain Research Station: 56-61.
Wasser, C.H. 1982. Ecology and culture of selected species useful in revegetating disturbed lands in the West. FWS/OBS-82/56. Washington, D.C.: U.S. Department of the Interior, Fish and Wildlife Service, Office of Biological Services, Western Energy and Land Use Team. 347 p.
Welch, B.L. 2004. Nutritive principles in restoration and management. In: Monsen, S.B.; Stevens, R.; Shaw, N.L., comps. Restoring western ranges and wildlands, vol. 1. Gen. Tech. Rep. RMRS-GTR-136-vol-1. Fort Collins, CO: U.S. Department of Agriculture, Forest Service, Rocky Mountain Research Station: 175-186.
Welsh, S.L.; Atwood, N.D.; Goodrich, S.; Higgins, L.C., eds. 2015. A Utah Flora. Fifth Edition, revised. Provo, UT: Brigham Young University. 990 p.
West, N.E. 1979. Survival patterns of major perennials in salt desert shrub communities of southwestern Utah. Journal of Range Management. 32(6): 442-445.
Whitacre, M.K.; Call, C.A. 2006. Recovery and germinability of native seed fed to cattle. Western North American Naturalist. 66(1): 121-128.
Young, J.A.; Young, C.G. 1986. Collecting, processing and germinating seeds of wildland plants. Portland, OR: Timber Press. 236 p.
Young, S.A.; Schrumpf, B.; Bouck, M.; Moore, M. 2020. How the Association of Official Seed Certifying Agencies (AOSCA) tracks wildland sourced seed and other plant propagating materials. Logan, UT: Utah State University, Utah Agricultural Experiment Station. 36 p.
How to Cite
Gucker, Corey L.; Shaw, Nancy L. 2024. Gooseberryleaf globemallow (Sphaeralcea grossulariifolia). In: Gucker, C.L.; Shaw, N.L., eds. Western forbs: Biology, ecology, and use in restoration. Reno, NV: Great Basin Fire Science Exchange. Online: https://westernforbs.org/