Authorship
Gucker, Corey; Shaw, Nancy
Publication Date
April 2023
Nomenclature
Pale agoseris (Agoseris glauca [Pursh] Raf.) belongs to the Asteraceae family, Cichorieae tribe, and Microseridinae subtribe (Feuer and Tomb 1977; Baird 2006).
Family
Asteraceae – Aster family
Genus
Agoseris
Species
glauca
NRCS Plant Code
AGGL (USDA NRCS 2022).
Subtaxa
The Flora of North America (Baird 2006) recognizes two varieties: A. g. var. glauca (Pursh) Raf. and A. g. var. dasycephala (Torrey & A. Gray) Jepson.
Synonyms
Troximon glaucum Pursh. (Baird 2006).
Synonyms for variety glauca: Agoseris lacera Greene, A. lapathifolia Greene, A. longissima Greene, A. longula Greene; A. microdonta Greene, A. procera Greene, A. vicinalis Greene (Baird 2006).
Synonyms for variety dasycephala: Troximon glaucum Pursh var. dasycephalum Torrey & A. Gray, A. altissima Rydberg, A. aspera (Rydberg) Rydberg, A. eisenhoweri B. Boivin, A. glauca subsp. aspera (Rydberg) Piper, A. glauca var. aspera (Rydberg) Cronquist, A. glauca var. pumila (Nuttall) Garrett, A. glauca subsp. scorzonerifolia (Schrader) Piper, A. glauca var. villosa (Rydberg) G. L. Wittrock, A. isomeris Greene, A. lanulosa Greene, A. leontodon Rydberg var. aspera Rydberg, A. leontodon var. pygmaea Rydberg, A. maculata Rydberg, A. pubescens Rydberg, A. pumila (Nuttall) Rydberg, A. scorzonerifolia (Schrader) Greene, A. turbinata Rydberg, A. vestita Greene, A. villosa Rydberg (Baird 2006).
Common Names
Pale agoseris, false dandelion, field agoseris, mountain dandelion, pale mountain dandelion, prairie agoseris, short agoseris (Baird 2006; LBJWC 2009; Welsh et al. 2016).
Chromosome Number
Chromosome numbers are: 2n = 18, 36. 2n = 18 for variety glauca; 2n = 36 for variety dasycephala (Baird 2006).
Hybridization
Hybridization is common within the Agoseris genus. It is especially common among polyploid taxa, and some hybrid populations are persistent (Baird 2006). Two hybrids have been described. Agoseris × agrestis Osterhout, an A. glauca var. glauca × A. parviflora hybrid, occurs in the foothills of Colorado’s Rocky Mountains. This hybrid has widely been referred to as A. glauca var. agrestis (Osterhout) Q. Jones, a name mistakenly applied to many specimens of A. glauca var. dasycephala. Agoseris × dasycarpa Greene, an A. glauca var. glauca × A. monticola hybrid, occurs primarily on the Modoc Plateau of northeastern California and adjacent Oregon (Baird 2006). Hybridization also occurs between variety dasycephala and A. aurantiaca and A. parviflora (Baird 2006).
Distribution
Pale agoseris is widely distributed throughout the western United States and Canada (Table 1). In the US, it occurs in all states west of Colorado and in the Dakotas, Minnesota, and Michigan (Baird 2006). Distributions of the two varieties overlap but variety glauca occurs farther south and variety dasycephala occurs farther north. In the states and provinces where their distributions overlap, variety glauca generally occupies lower elevation sites and variety dasycephala higher elevation sites (Hitchcock and Cronquist 2018). The varieties become difficult to distinguish in the northern part of the species range (e.g., southeastern British Columbia and southwestern Alberta), where populations occur near one another and there are pockets of complete introgression (Baird 2006).
Table 1. States and provinces where pale agoseris varieties occur (Baird 2006).
States | Variety glauca | Variety dasycephala |
Arizona | X | |
California | X | |
Colorado | X | X |
Idaho | X | X |
Michigan | X | |
Minnesota | X | |
Montana | X | X |
Nevada | X | |
New Mexico | X | |
North Dakota | X | X |
Oregon | X | X |
South Dakota | X | X |
Utah | X | X |
Washington | X | X |
Wyoming | X | X |
Provinces and Territories | ||
Alberta | X | X |
British Columbia | X | X |
Manitoba | X | X |
Northwest Territories | X | |
Ontario | X | |
Saskatchewan | X | X |
Yukon | X |
Habitat And Plant Associations
As its broad distribution and wide elevation range suggest, pale agoseris grows in a wide variety of habitats from valley grasslands to montane forests and alpine communities. It grows on any aspect in dry to moist or even wet conditions in meadows, sagebrush (Artemisia spp.) steppe (Fig. 1), pinyon-juniper (Pinus-Juniperus spp.) woodlands, coniferous forests, and alpine tundra (Craighead et al. 1963; Beatley 1976; Currah et al. 1983; Hickman 1993; Cronquist et al. 1994; Mansfield 2000; Schiebout et al. 2008; Lesica 2012; Welsh et al. 2016). Plants are typically scattered but can be abundant on disturbed sites and in upland rangeland vegetation (Sampson 1917; Currah et al. 1983). Common associates in Utah include big sagebrush (A. tridentata), smooth brome (Bromus inermis), and mule-ears (Wyethia spp.) (USU Extension 2017).
Figure 1. Pale agoseris in flower growing with sagebrush. Photo: USDA USFS Provo Shrub Science Laboratory.
Pale agoseris grows in a variety of grassland and shrubland types. Plants occurred in both the willow (Salix spp.) and Altai fescue (Festuca altaica) interspaces of quaking aspen (Populus tremuloides) woodlands in Alberta’s Rocky Mountains 68 miles (110 km) north of the US border. Frequency of pale agoseris was very similar in grass- and shrub-dominated vegetation (Hersperger and Forman 2003). Hurd (1961) and Despain (1973) described pale agoseris as a major associate of mid- to high-elevation Idaho fescue (Festuca idahoensis) communities in Wyoming’s Big Horn Mountains. Pale agoseris occurred in several mountain big sagebrush (A. tridentata subsp. vaseyana) and mountain snowberry (Symphoricarpos oreophilus) shrublands when Tueller and Eckert (1987) studied these associations in northern Nevada. It was common in several sagebrush communities when 372 late-seral shrublands were studied in the Humboldt National Forest at 5,900–10,200 ft (1,800–3100 m) with mean annual precipitation of 13 in (330 mm). In the mountains of northeastern Nevada, constancy of pale agoseris was 75% in little sagebrush (A. arbuscula subsp. longiloba)/Idaho fescue habitats at 6,001–6,499 ft (1829–1981 m) elevation with mean soil depth of 32 in [81 cm]), 50% in mountain big sagebrush/bluebunch wheatgrass (Pseudoroegneria spicata) habitats at 5,600–8,999 ft (1,707–2,743 m) elevation with mean soil depth of 44 in (111 cm), and 48% in mountain big sagebrush/mountain snowberry/bluebunch wheatgrass at 6,601–8,999 ft (2,012–2,743 m) elevation with mean soil depth of 46 in (117 cm) (Jensen et al. 1988).
Pale agoseris is also described in several woodland and forest communities. In the Upper Walker River in California and Nevada, plants occurred in low- and high-elevation pinyon-juniper woodlands (Fig. 2), Jeffrey pine-white fir (Pinus jeffreyi–Abies concolor) forests, lodgepole pine (Pinus contorta) stands, whitebark pine (P. albicaulis) communities, and in the alpine zone (Lavin 1983). In Wyoming’s Jackson Hole Wildlife Park, pale agoseris was common in sedge-grass meadows, abundant in sagebrush shrublands, and present in lodgepole pine understories (Reed 1952). Plants were well-developed in the understory of lodgepole pine/Wheeler bluegrass (Poa nervosa) habitat types where the forest floor was open and dry at 7,900 to 9,500 ft (2,400–2,900 m) elevation in Wyoming’s Wind River Mountains (Reed 1976). Throughout Red Butte Canyon near Salt Lake Valley, Utah, plants were common in fringe vegetation surrounding Gambel oak (Quercus gambelii) woodlands (Ehleringer et al. 1992). When understory communities in montane and subalpine forests were compared on the North Rim of the Grand Canyon in Arizona, pale agoseris was an indicator species in montane zone ponderosa pine (P. ponderosa) and ponderosa pine-Gambel oak forests (Laughlin et al. 2005). A variety of habitats were occuppied in the Sangre de Cristo Mountains of Santa Fe County, New Mexico. Pale agoseris occurred at elevations of 7,050 to 12,450 ft (2,150–3,800 m) in montane meadows, Engelmann spruce-corkbark fir (Picea engelmanii–Abies lasiocarpa var. arizonica) forests, alpine meadows, and burned areas (Larson et al. 2014).
Figure 2. Pale agoseris setting seed in a sagebrush and juniper community in California. Photo: USDI BLM CA370 SOS.
Pale agoseris is common in several subalpine and alpine vegetation types. In an analysis of 209 alpine stands in the North Cascade Mountains of Washington and British Columbia, pale agoseris was most common in greenleaf fescue (F. viridula) communities. These communities occurred at 6,070 to 7,050 ft (1,850–2,150 m) elevation on well-drained south slopes where snow melts in late May or early June (Douglas and Bliss 1977). When above tree line vegetation was studied on Mount Washburn in Yellowstone National Park, Wyoming, pale agoseris was most constant in Payson’s sedge-alpine sagebrush (Carex paysonis–Artemisia scopulorum) communities (Aho and Weaver 2010). In a more extensive evaluation of the same area, pale agoseris had broad altitudinal distribution. It was present at the highest altitudes and far below treeline, in dry to wet soils, and in all habitats including disturbed (Aho and Bala 2012). In surveys of alpine vegetation on the White Cloud Peaks in Custer County, Idaho, cover of pale agoseris was 2.3% in Gordon’s ivesia (Ivesia gordonii)-dominated fell fields, 2% in field sagewort (Artemisia campestris) communities, 7.1% in cushion phlox (Phlox pulvinata) communities, and 1.8% cover in dry, meadowlike turf communities (Richardson and Henderson 1976). Pale agoseris was associated with tufted hairgrass (Deschampsia cespitosa) meadows where snow melt is late on Wheeler Peak in northern New Mexico (Baker 1983).
Elevation
Pale agoseris has been reported to grow at all elevations within its range (Craighead et al. 1963; Lesica 2012). Populations are described from 300 to 12,500 feet (90–3,810 m) (Hickman 1993; Cronquist et al. 1994; Baird 2006; Blackwell 2006) although the Flora of North America indicates an elevation range of 300–10,800 ft (90–3,300 m) for variety glauca and a slightly broader range of 300 to 11,800 ft (100–3,600 m) for variety dasycephala (Baird 2006). The elevation range for the species is reported as 4,600–12,500 ft (1,400-3,800 m) in California (Hickman 1993) and 4,300 to 12,000 ft (1,300–3,660 m) in Utah (Welsh et al. 2016).
Soils
Pale agoseris is considered very adaptable, growing in many variations of soils and moisture conditions (USFS 1937). Density and size of plants can be greatest on open plateaus and well-drained glades with loose, gravelly, deep, clay loams (Sampson 1917). Well-drained, nutrient-poor, sandy to gravelly soils are described in pale agoseris habitats (USU Extension 2017; PFAF 2022). Variety glauca is common in wet meadows, on stream margins, swales, and other moist sites with slightly alkaline or saline silts, clays, and fine-textured soils. Variety dasycephala is common in dry to moist gravelly, rocky, and other coarse-textured soils (Fig. 3) (Baird 2006).
Figure 3. Pale agoseris setting seed at a rocky site in Oregon. Photo: USDI BLM OR010 SOS.
Parent material/texture. Parent material and texture of soils were described in communities where pale agoseris is common in Idaho and Wyoming. In surveys of the alpine vegetation on the White Cloud Peaks in Custer County, Idaho, cover of pale agoseris was 7.1% in cushion phlox communities found on mostly flat to steep slopes on sedimentary deposits (Richardson and Henderson 1976). Cover was 2.3% in Gordon’s ivesia-dominated fell fields with poor to moderately developed soils of gravelly, glacial sedimentary rock deposits. Cover was 2% in field sagewort communities on sedimentary deposits with shallow, rocky, poorly developed soils. Cover was 1.8% in dry, meadowlike turf habitats with extensive soil development and high plant cover (Richardson and Henderson 1976). Pale agoseris was a common forb (100% constancy and 74% frequency) in nine Idaho fescue grassland stands studied in the Big Horn National Forest in north-central Wyoming. Soil parent materials were granite, sandstone shale, or limestone and ranged from clay to sandy loams (Hurd 1961).
Moisture/temperature. Pale agoseris grows in soils described as xeric to wet-mesic. An early US Rangelands guidebook describes pale agoseris as fairly drought tolerant with plants not wilting excessively in finely disintegrated soils until soil-water content is reduced to 8 to 10% (Sampson 1917). Pale agoseris was described as an indicator of xeric stands in an analysis of 127 alpine larch (Larix lyallii) stands evaluated across the range of alpine larch (6,300–9,600 ft [1,920–2,925 m]) (Arno and Habeck 1972). In the alpine and subalpine regions of Helm Valley, southwestern British Columbia, pale agoseris occurred in deep, loose cinder soils where snow melts early in the season and insolation and surface soil temperatures were high at times (Brink 1964). In a study of fescue grasslands in western Canada, pale agoseris was considered an indicator of mesic to wet-mesic conditions in rough fescue-shortbristle needle and thread (F. campestris–Stipa spartea var. curtiseta) communities (Looman 1969). In subalpine meadows in Utah’s Unita Mountains on April 1, pale agoseris cover was greatest (0.5%) in the light snowpack zone (water content: 16.8 in [42.7 cm]) and lower (0.1%) in the moderate snowpack zone (22.4 in [56.9 cm]) and heavy snowpack zone (31.5 in [80.1 cm]) (Ostler et al. 1982). On Mt Washburn in Yellowstone National Park, pale agoseris was most constant in Payson’s sedge-alpine sagebrush vegetation in the alpine zone. Of the six alpine vegetation types compared, this community had the second highest water availability, lowest observed maximum soil temperature, and a frost-free season averaging 93 days (Aho and Weaver 2010).
Depth. Pale agoseris tolerates a variety of soil depths. On Steens Mountain in Oregon, it is described as growing in dry, shallow, gravelly soils (Mansfield 2000). When 10 Idaho fescue subalpine meadows northeast of Bozeman, Montana, were compared, pale agoseris constancy and frequency were similar across all meadows (Weaver 1974). Plants showed no preference for soil depth or snow melt date. Soil depth ranged from 6 to 18+ in (15–45+ cm) and snowmelt dates ranged from before May 22 to July 15. Diversity, cover, and productivity of the meadows decreased with decreased soil depths and later snow melt out days (Weaver 1974).
Description
Distinguishing Agoseris species is difficult because traits can vary region to region. Misleading and inaccurate descriptions exist in the literature, and hybridization and intermediate forms are common (Baird 2006). Although many variant forms exist, pale agoseris is perennial and scapose with solitary, terminal yellow flowerheads and milky sap (Fig. 4) (Sampson 1917; Stubbendieck et al. 1986; Taylor 1992; LBJWC 2009). Plants have slender, erect stems that can be 30 in (80 cm) tall in areas with long growing seasons (Sampson 1917; Taylor 1992; USU Extension 2017). They produce strong and often deep taproots and strong, simple to branching caudices (Sampson 1917; Wynd 1936; USFS 1937; Andersen and Holmgren 1996; Welsh et al. 2016). In loose soils, the taproot is sparsely branched and can penetrate 2 ft (0.6 m), but in rocky soils the taproot is often unbranched (Sampson 1917).
Figure 4. Pale agoseris plant with one flowering stem and one stem with developing seeds. Photo: USDI BLM WY020 SOS.
All leaves are basal. They are erect to decumbent, nearly lanceolate to oblanceolate, and range from 0.8 to 18 in (2–46 cm) long. Leaf margins are typically entire but sometimes dentate and rarely lobed (Baird 2006). Leaves are up to half as long as the flowering stalks (Sampson 1917; USU Extension 2017). Leaf surfaces are glaucous or not and range from glabrous to villous pubescent with a distinct midvein (Fig. 5) (Stubbendieck et al. 1986; Welsh et al. 2016).
Figure 5. Entire, lanceolate basal pale agoseris leaf with a distinct midvein. Photo: USDI BLM WY020 SOS.
Flowerheads are perfect, flat-topped, and up to 2 in (5 cm) across when open (Stubbendieck et al. 1986; Luna et al. 2018). They include 15 to 150 ligulate florets (Baird 2006). Peduncles are generally glabrous or glabrate, elongate after flowering, and typically range from 2 to 24 in (5–60 cm) in fruit (Fig. 6) (Baird 2006). Involucres are obconic to hemispheric and 0.4 to 1 in (1–3 cm) tall. Phyllaries occur in two or three subequal to unequal series and are green or medially rosy purple, often with purple-black spots (Stubbendieck et al. 1986; Baird 2006; Welsh et al. 2016). Plants produce one-seeded achene or cypselae fruits, referred to as seeds. Seeds are fusiform to narrowly conic and 5 to 9 mm long with stout tapered beaks that are 1 to 4 mm long or about half as long as the seed body (Cronquist 1994; Baird 2006; Welsh et al. 2016). Seeds are glabrous to distally scabrous with flattened to ridged ribs. Wind-dispersal is facilitated by the pappus with two to three series bristles that is 0.3 to 0.7 in (8–18 mm) long (Taylor 1992; Baird 2006).
Figure 6. Pale agoseris plant with one fading flowerhead and one seed head. Photo: USDI BLM OR931 SOS.
Variety glauca generally produces leaves with entire, straight margins. Leaf faces can be glaucous and glabrous to sparsely hairy (Baird 2006). Its are usually glabrous but are sometimes apically puberulent, and phyllaries are usually green but can be medially rose-purple and are often purple-black spotted or tipped. Variety dasycephala generally produces leaves that are glabrous to densely villous adaxially with undulate margins or lobes. Its peduncles are usually apically villous or sometimes stipitate-glandular. Phyllaries are often medially rose-purple or rarely all green, often with some combination of purple-black spots, speckles, midstripes, or tips (Baird 2006).
Reproduction
Pale agoseris reproduces from seed, and insect pollination is required for seed production (Jensen 2008).
Phenology
Pale agoseris flowering can occur from May to September (Baird 2006), although other sources suggest that flowers are most common in May and June (Currah et al. 1983; Stubbendieck et al. 1986; USU Ext 2017; PFAF 2022). Flowers appear later at high-elevation sites (Craighead et al. 1963). Variety glauca exhibits a longer flowering period (May-September) than variety dasycephala (July and August) (Baird 2006). Mature seed ripens from June to September (Currah et al. 1983; USU Ext 2017; PFAF 2022).
Pollination
Flowers are pollinated by a variety of bees, wasps, flies, and butterflies (Burkle and Irwin 2009). Pale agoseris flowers in high-elevation meadows 9,500 ft (2,900 m) near Crested Butte, Colorado, were visited by pollinators from the following families: Andrenidae, Anthomyiidae, Apidae, Bombyliidae, Colletidae, Halictidae, Ichneumonidae, Muscidae, Nymphalidae, Rhagionidae, Sarcophagidae, and Syrphidae. Flower-pollinator interactions were observed in good weather conditions from 9 am to 4 pm from June through August over three years (Burkle and Irwin 2009). In a field experiment conducted in a subalpine meadow in Gunnison County, Colorado, pale agoseris flowers were open and available only in the morning. Flowers were visited by a variety of bumblebees including Bombus silvicola, B. frigidus, B. occidentalis, and B. flavifrons (Thomson 1981). Bee fauna and flora relationships were studied from shrub steppe to high alpine communities in north-central Washington’s Okanogan-Wenatchee National Forest. Bumblebees (Bomus bifarius, B. insularis), leafcutting bees (Coelioxys sodalist), furrow bees (Halictus farinosus, H. annulatus), sweat bees (Lasioglossum spp.), and mason bees (Osmia brevis, O. coloradensis, O. tristella) visited pale agoseris flowers (Wilson et al. 2010).
Ecology
Pale agoseris is a common short-lived species (Eldredge et al. 2013) that can spread rapidly on disturbed sites (Currah et al. 1983) but also grows in open spaces of early to late-seral communities (Evenson et al. 1980; Allen and Nowak 2008). Large mats of plants of up to 60 in (150 cm) in diameter have been reported on disturbed sites, but these are rarely as large on undisturbed sites (Currah et al. 1983).
When habitats in southwestern Washington were visited 13 to 14 years after the eruption of Mount Saint Helens, pale agoseris occurred in many primary successional communities. It was one of many wind-dispersed Asteraceae colonizers (Titus et al. 1998).
In the understories of ponderosa pine, Gambel oak, Rocky Mountain juniper (Juniperus scopulorum), and snowbrush ceanothus (Ceanothus velutinus) communities near Kamas, Utah, pale agoseris occurred more often in canopy openings where light intensity was greatest and litter depth and soil depth were least (Evenson et al. 1980). When nine singleleaf pinyon-Utah juniper (Pinus monophylla–Juniperus osteosperma) woodland stands with various amounts of tree cover were compared in central Nevada’s Shoshone Mountain Range, pale agoseris was present with very low cover in two of the low and one of the medium tree cover plots (Allen and Nowak 2008).
Seed And Seedling Ecology
Pale agoseris produces numerous seeds adapted for wind dispersal (Fig. 7) (Sampson 1917). Seed production of a single mature pale agoseris plant of average size and growing with low competition in North Dakota was 34.25 seeds/seed head or capitulum (Stevens 1957). Seed fill can be limited by predation (Currah et al. 1983), which varies by location (Johnson 2008).
Figure 7. Pale agoseris seed head fully opened. Photo: USDI BLM OR931 SOS.
Seed damage from predation ranged from 0 to 100% but averaged only 8% when three populations of pale agoseris in central Utah were examined in 2006 and 2007. Plants produced an average of 38 seeds/capitulum (range 12–73 seeds/capitulum). Seed production differed significantly by site (P < 0.01) but not by year, suggesting differences were related to environmental or genetic factors. Average seed production was lowest at Manti Ridge (33.6 seeds/capitulum) and highest at Willow Creek (41.8 seeds/capitulum) (Johnson 2008).
In a field experiment in a native grassland in Alberta, Canada, protection of pale agoseris seedling roots from neighboring plant roots improved survival but did not impact biomass accumulation (Cahill 2003). Seedlings were transplanted with or without root exclusion pipes (6 in [15 cm] diameter and 8 in [20 cm] long) within intact vegetation in early May. Seedlings were watered daily for the first week after transplanting. Effects of root protection were evaluated on August 20. Pale agoseris seedling mortality was double for seedlings without root protection (60%) than for seedlings with roots protection (30%), but the differences were not significant (P > 0.2). Biomass of surviving plants and relative allocation to root biomass was not affected by root protection treatments (Cahill 2003).
Seed and seedling fates of pale agoseris were examined in the alpine zone of the Beartooth Plateau in Montana (Chambers 1995). The study site had sparse vegetation cover (<25%), sandy loam soil, an average growing-season precipitation of 5 in (131 mm), and wind that averaged (11 ft [3.4 m]/s at 16 in [40 cm]) with gusts up to 75 ft (23 m)/s. Pale agoseris seeds at this site averaged 0.8 in (2.1 cm) long including the pappi. When <0.5 to 16 mm particle size seed traps were compared, more seeds were trapped in soils with 4 to 8 mm particle size. Pale agoseris seedling emergence was low and limited to traps with <0.5, 1 to 2, and 2 to 4 mm particles. Few seedlings survived to the end of the growing season. In mixed particle size soil seed traps, higher numbers of pale agoseris seeds were trapped in the predominantly coarse-particle size soils than in fine or intermediate, but seedling survival was greatest (although still low, <15%) in predominantly fine size soil particles (Chambers 1995).
Disturbance Ecology
Pale agoseris abundance was often greater on disturbed than on undisturbed plots in ecological studies of grazing, logging, and fire (see below). The USFS (USFS 1937) suggests that long taproots and good reproductive powers enable it to survive in disturbed and eroded conditions.
Pale agoseris density increased least on protected sites in a study comparing vegetation of a mixed grass-forb meadow grazed by sheep and/or gophers (Thomomys spp.) (Branson and Payne 1958). Density was compared on plots in the Bridger Mountains of Montana. Plots were heavily grazed by sheep until 1929 then moderately grazed until 1956. Treatments to compare the effects of sheep and gopher grazing were initiated in 1943. Changes in the density of pale agoseris between 1951 and 1956 were +54 stems on plots closed to both sheep and gophers, +80 stems in plots open to both sheep and gophers, +148 stems on plots closed to sheep but open to gophers, and +415 stems on plots open to sheep but closed to gophers (Branson and Payne 1958). Similarly, Vaughan (1974) found that pale agoseris was most typical of dry sites or sites disturbed pocket gopher mound construction in a study of small mammals in subalpine meadows of northern Colorado (Vaughan 1974).
Cover of pale agoseris was greater in cut and burned than cut and unburned treatments in a 90-year-old western juniper (Juniperus occidentalis) woodland on Steens Mountain in southeastern Oregon. Treatment differences in pale agoseris cover were only significant in the first three to four years of treatments (Bates and Svejcar 2009).
In the subalpine zone north of Jasper National Park in Alberta, Canada, pale agoseris was more abundant on burned than on unburned plots within a study area. The area was prescribed burned in 1970 and post-fire conditions were evaluated in 1980. The prominence value (a product of average cover and the square root of average frequency) for pale agoseris was 17.4 on burned plots and 1.9 on unburned plots (Bentz and Woodard 1988). When sites burned 1 to 14 years earlier were compared in mountain big sagebrush communities in southeastern Idaho, pale agoseris was characteristic of the most recently burned plots. Abundance of pale agoseris started to decrease on plots burned 6 to 14 years earlier (Nelle et al. 2000). Pale agoseris frequency was lower on burned than unburned plots the year following an “intense” August fire near Midway, Utah. The burned area included Gambel oak thickets intermixed with big sagebrush-grass vegetation. Frequency of pale agoseris averaged 9.1% in unburned and 0% in unburned shrub-grass and 11.8% in unburned and 0.9% in burned Gambel oak thickets (Poreda and Wullstein 1994).
Wildlife And Livestock Use
Pale agoseris is an important wildlife and livestock species. It is a food source for native ungulates, sheep, cattle, a variety of small mammals, birds, and many insect species.
Native ungulates. Pale agoseris is considered desirable forage for elk (Cervus canadensis), deer (Odocoileus spp.), pronghorn (Antilocapra americana), sheep, and horses in spring and summer, and cattle in the spring (Ogle and Brazee 2009). Several studies show that pale agoseris is an important spring and summer food for native ungulates. A review of elk food habits labeled pale agoseris a highly valuable summer elk food (Kufeld 1973). In elk feeding observations made in the Gallatin Drainage in Montana, pale agoseris was among the three most often eaten forbs (Brazda 1953). Elk were also observed feeding on pale agoseris in May and June in Montana’s Sun River Drainage (Knight 1970).
Mule deer (Odocoileus hemionus) also feed on pale agoseris. It was 4% of observed instances of mule deer summer use in the Bridger Mountains of Montana (Wilkins 1957). When captive mule deer were fed rangeland forage collected near Logan, Utah, pale agoseris was eaten in early summer (May 1-June 20) but not after that time. Feeding trials ended on September 30 (Smith 1953). On the Kaibab Plateau in Arizona, mule deer feeding on summer range was evaluated by the feeding minutes method. Pale agoseris was important and made up 3.7% of total feeding time and a high of 12% feeding time from June 1 to June 15 (Hungerford 1970).
Pale agoseris made up a large proportion of elk and sheep diets when range use in the Crow Creek drainage of Montana’s Elkhorn Mountain was compared for elk, cattle, and sheep. Feeding observations were made for two years in Idaho fescue-bluebunch wheatgrass, spruce-fir (Picea–Abies spp.), and Douglas-fir (Pseudotsuga menziesii) communities. Pale agoseris constituted 14% of sheep and 9% of elk diets (Stevens 1966).
Livestock. Pale agoseris is considered fair to good sheep forage and is lightly grazed by cattle and horses. Plant abundance tends to decrease with sheep grazing and increase with cattle grazing (Stubbendieck et al. 1986). On mountain summer ranges in Utah, average percent use of pale agoseris was 52% by sheep and 2% by cattle (Cook 1983). Sheep fed on pale agoseris multiple times a year, especially in years where summer conditions allowed leaves to stay green (USFS 1937). Sampson (1917) reported that sheep seek out pale agoseris and prefer it to many grasses and other forbs. Each season they will eat plants to the ground. They graze leaves, flower stalks, and flowers until seeds are nearly mature (Sampson 1917).
Effects of long-term sheep grazing on high-elevation summer range in southwestern Utah also suggest that pale agoseris is preferred. An area that was largely protected from sheep and used only minimally by cattle and horses for about 30 years averaged 66 lbs/ac (74 kg/ha) of pale agoseris aboveground biomass. Pale agoseris plants were lacking in the sheep-grazed area (Bowns and Bagley 1986).
In studies that compared methods for estimating sheep diets, pale agoseris made up 3 to 10% of diets (Laycock 1967; McInnis et al. 1983). In a dry meadow in eastern Oregon, pale agoseris was 3.7% of diets by the forage utilization method but a trace to none by the esophageal fistulation, stomach content analysis, and fecal analysis diet estimation methods (McInnis et al. 1983). In a tall forb summer range (7,800 ft [2,400 m]) on the US Sheep Experiment Station in southeastern Idaho, pale agoseris was 6 to 10% of sheep diets when using fistula, clipped, and ocular methods of estimating diets (Laycock 1967).
Cattle preference for pale agoseris was high when utilization of a grass-forb summer range was evaluated over four years in Wyoming’s Big Horn Mountains. Pale agoseris together with silky lupine (Lupinus sericeus), white locoweed (Oxytropis sericea), and dandelion (Taraxacum officinale) produced 86% of the forb forage (Hurd and Pond 1958).
A variety of small mammals feed on pale agoseris leaves, roots, and seeds. Cliff chipmunks (Tamias dorsalis) fed heavily on stems, flowers, and seeds while rock squirrels (Spermophilus variegatus) primarily fed on the leaves of pale agoseris in Cache County, Utah (Hart cited in Hart 1992). Pale agoseris was the second most preferred of 10 species presented to Belding’s ground squirrel (Spermophilus beldingi) in cafeteria-style feeding experiments. Animals were captured from mountain meadows in the Carson Range in the Sierra Nevada Mountains of western Nevada. Leaves were eaten more than flowers, and flowers were eaten more than stems (Eshelman and Jenkins 1989). In other cafeteria-style experiments, food selection by mountain pocket gopher (Thomomys monticola) was evaluated for animals trapped from a montane meadow on the east side of the Sierra Nevada Mountains near Verdi, Nevada. Roots of each species ground into a powdered form were presented as pellets. Two of the three gophers in the study selected pale agoseris root pellets significantly (P < 0.05) more than those of most other plants (Jenkins and Bollinger 1989). In two summers of observations near West Yellowstone National Park in Gallatin County, Montana, male western chipmunks (Eutamias minimus and amoenus) extended portions of their ranges in June, likely in search of pale agoseris. They were continually found feeding on pale agoseris seeds, and in the study area, pale agoseris plants were sparsely distributed and widely scattered (Martinsen 1968). In studies conducted in subalpine meadows in northern Colorado, pale agoseris averaged 12% of northern pocket gophers (T. talpoides) summer diets in 1965 and 4.2% in 1966. It averaged 14.6% in montane vole diets in 1965 (Vaughan 1974). In analysis of stomach contents of western jumping mouse (Zapus princeps) from northern Colorado, pale agoseris leaves made up 2.3% of July and 1.9% of August diets. Forb seeds were also recovered, but they were not identified to species (Vaughan and Weil 1980).
Game birds. Pale agoseris plants and associated insects are important to greater sage-grouse. The leaves of pale agoseris are eaten by greater sage-grouse (Centrocercus urophasianus) in the late spring and early summer brood-rearing season (Luna et al. 2018). Agoseris spp. were one of three forbs with consistently high selection values when the habitat relationships of pre-laying greater sage-grouse hens were studied in southeastern Oregon. It was a primary diet component and its frequency in hen crops ranged from 11% to 69%. (Barnett and Crawford 1994). In a review of forbs and their importance to greater sage-grouse, Agoseris spp. were listed as important spring foods for female greater sage-grouse and they provided food for adults and juveniles in summer (Pennington et al. 2016).
Pale agoseris is an important nectar source for a variety of insects (Hansen and Ueckert 1970; Watt et al. 1974; LaBerge 1989). Mormon fritillary butterflies (Speyeria mormonia) fed on Agoseris spp., among others, in a montane, temperate zone grassland at 9,680 ft (2,950 m) elevation near Gunnison County, Colorado. Pale agoseris flowers produced nectar concentrations of 37% sucrose (Watt et al. 1974), which was within the range of concentrations producing the maximum rate of sugar intake for Mormon fritillary (Boggs 1988). In nectar-feeding surveys conducted in subalpine meadows at Jumpingpound Ridge in Alberta, pale agoseris flowers were preferred by butterflies. In 214 nectar-feeding events observed on 19 species of flowers over three summers, feeding on pale agoseris flowers was observed third most among all forbs (18 times) for western whites (Pontia occidentalis), most of all species for clouded sulphur (Colias philodice) and Labrador sulphur (C. nastes). Feeding was observed but was less by cabbage whites (Pieris rapae) and Mead’s sulphur (C. meadii) (Ezzeddine and Matter 2008). Pale agoseris was also eaten by red-shanked grasshoppers (Xanthippus corallipes) and Mormon crickets (Anabrus simplex), although not preferred in the mountain herbland studied in Roosevelt National Forest in Larimer County, Colorado (Hansen and Ueckert 1970). Many bees species have been collected from pale agoseris flowers including death camas miner bees (Andrena astragali) (Laberge and Ribble 1975), parsnip miner bees (A. thaspii), Buckell’s miner bees (A. buckelli) (LaBerge 1980), and pale-faced miner bees (A. pallidifovea) (LaBerge 1989). More bee visitors are discussed in the Pollination section above.
Nutritional Value
The value of pale agoseris forage was evaluated at three sites in studies related to ungulates, small mammals, and game birds. The gross energy value of aboveground pale agoseris averaged 4.35 kcal/g from mid-July to the end of August biweekly sampling in alpine vegetation (10,600 ft [3,200 m]) in the Snowy Range of Wyoming (Smith 1967). A study involving the use of pale agoseris by small mammals, found that pale agoseris had high water content and flowers and leaves provided more protein than stems (Table 2; Eshelman and Jenkins 1989). In habitats used by greater sage-grouse in southeastern Oregon, Agoseris spp. leaves averaged 26% crude protein, 0.44% calcium, and 0.48% phosphorus (Barnett and Crawford 1994).
Table 2. Nutrient content of pale agoseris plants collected from mountain meadows in the Carson Range of the central Sierra Nevada Mountains in western Nevada (Eshelman and Jenkins 1989).
Plant part | Water (%) | Protein (%) | Crude fiber (%) | Fat (%) | Kilojoules/g |
Flowers | 78 | 14.2 | —- | —- | 20.2 |
Leaves | 75 | 11.0 | 14.3 | 5.3 | 18.4 |
Stems | 78 | 5.1 | —- | —- | 18.2 |
Ethnobotany
Pale agoseris plants contain a milky latex that is released when stems and leaves are broken. This latex was chewed like gum by the Thompson, Okanagan-Colville, and Spokane people. The Thompson also used the latex to remove warts (Turner et al. 1990). The Okanagan-Colville used an infusion of the entire plant to wash sores and rashes, a poultice of the latex to treat sores, and an infusion of roots as a laxative (Turner 1980 cited in Moerman 2003).
Revegetation Use
Pale agoseris has several traits that make it a good choice for revegetation. It thrives and spreads in disturbed sites and tolerates a range of soil types and moisture conditons (USFS 1937; Currah et al. 1983). It is recommended for pollinator habitat (Eldredge et al. 2013).
Developing A Seed Supply
For restoration to be successful, the right seed needs to be planted in the right place at the right time. Coordinated planning and cooperation is required among all partners to select appropriate species and seed sources; these are determined by current or projected revegetation requirements and goals (PCA 2015).
Developing a seed supply begins with seed collection from native stands. Collection sites are determined by current or projected revegetation requirements and goals as well as through consultation with land managers and stakeholders. Collected seed is distributed for restoration or first increased in seed fields to provide a larger supply of seed (PCA 2015). Production of nursery stock requires less seed than large-scale seeding operations. Regardless of the size and complexity of any revegetation effort, seed certification is essential for tracking seed origin from collection through use (UCIA 2015).
Seed Sourcing
Because empirical seed zones are not currently available for pale agoseris, generalized provisional seed zones developed by Bower et al. (2014) may be used to select and deploy seed sources. These provisional seed zones identify areas of climatic similarity with comparable winter minimum temperature and aridity (annual heat to moisture index). In Figure 8, Omernik Level III Ecoregions (Omernik 1987) overlay the provisional seed zones to identify climatically similar but ecologically different areas. For site-specific disturbance regimes and restoration objectives, seed collection locations within a seed zone and ecoregion may be further limited by elevation, soil type, or other factors.
The Western Wildland Environmental Threat Assessment Center’s (USDA FS WWETAC 2017) Threat and Resource Mapping (TRM) Seed Zone application provides links to interactive mapping features useful for seed collection and deployment planning. The Climate Smart Restoration Tool (Richardson et al. 2020) can also guide revegetation planning, seed collection, and seed deployment, particularly when addressing climate change considerations.
Occurrence Map
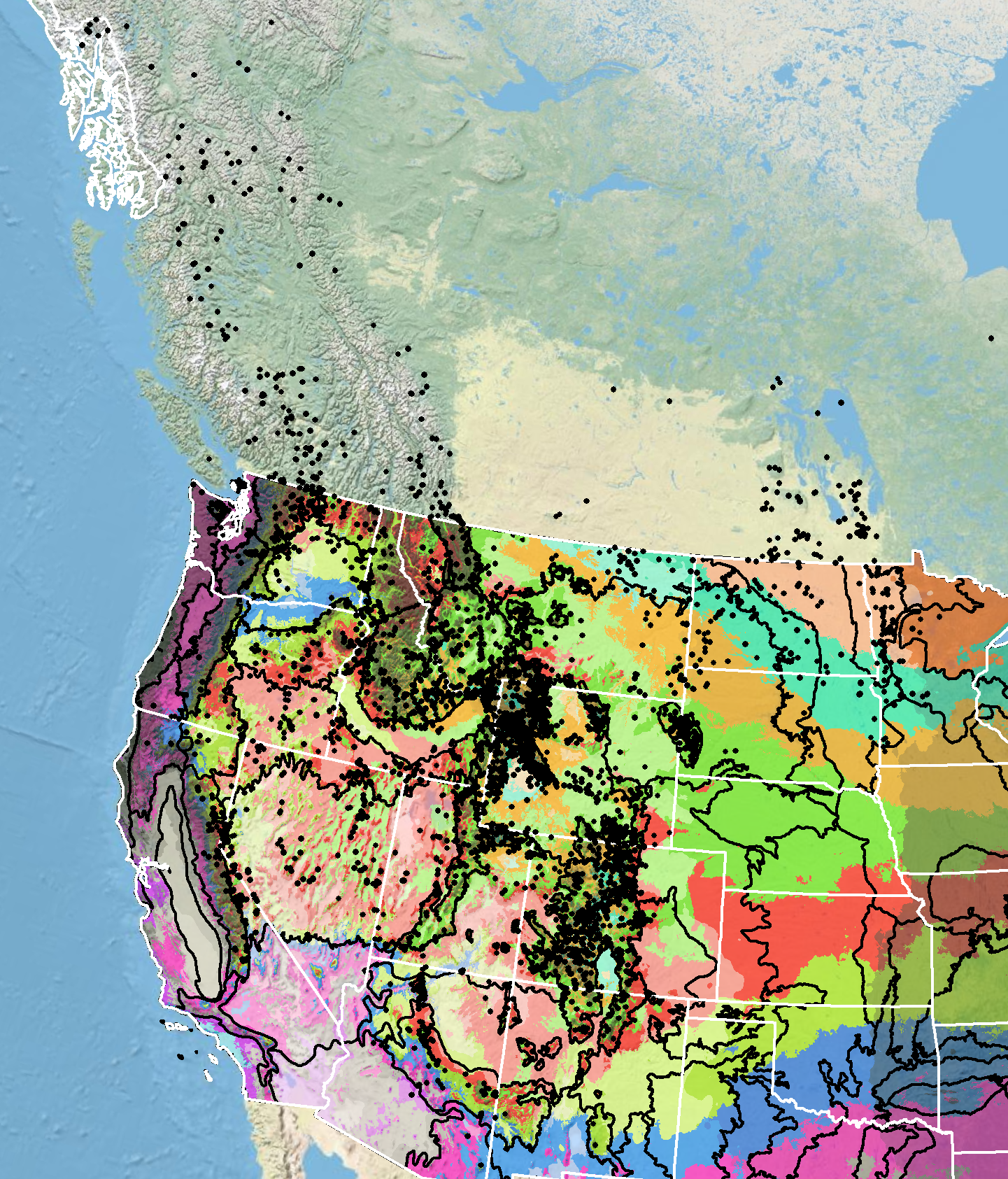
Figure 8. Distribution of pale agoseris (black circles) based on geo-referenced herbarium specimens and observational data from 1881–2016 (CPNWH 2023; SEINet 2023; USDI USGS 2020). Generalized provisional seed zones (colored regions) (Bower et al. 2014) are overlain by Omernik Level III Ecoregions (black outlines) (Omernik 1987; USDI EPA 2018). Interactive maps, legends, and a mobile app are available (USDA FS WWETAC 2017; www.fs.fed.us/wwetac/threat-map/TRMSeedZoneMapper2.php?). Map prepared by M. Fisk, USDI USGS.
Releases
As of 2023, there were no pale agoseris germplasm releases.
Wildland Seed Collection
Jensen (2005) summarizes the challenges with collecting wildland pale agoseris seed. The species can be difficult to locate as populations are most easily observed when plants are in full flower, but plants are often small, occur in low abundance, and are intermixed with larger stature species. The flowering season can be short, and flowering is not guaranteed annually. Seed retention time is short once seeds are mature and seed is readily wind dispersed (Jensen 2005). Currah et al. (1983) reports that although seed heads produce many seeds, seed fill can be low.
Wildland Seed Certification
Verification of species and tracking of geographic source is necessary whether wildland seed is collected for immediate project use or as stock seed for cultivated increase. This official Source Identification process can be accomplished by following procedures established by the Association of Official Seed Certifying Agencies (AOSCA) Pre-Variety Germplasm Program (UCIA 2015; Young et al. 2020). Wildland seed collectors should become acquainted with state certification agency procedures, regulations, and deadlines in the states where they collect.
If wildland-collected seed is to be sold for direct use in ecological restoration projects, collectors must apply for Source-Identified certification prior to making collections. Pre-collection applications, site inspections, and species and seed amount verification are handled by the AOSCA member state agency where seed collections will be made (see listings at AOSCA.org).
If wildland seed collected by a grower or private collector is to be used as stock seed for planting cultivated seed fields or for nursery propagation (See Agricultural Seed Field Certification section), detailed information regarding the collection site and collecting procedures must be provided when applying for certification. Photos and herbarium specimens may be required. Germplasm accessions acquired within established protocols of recognized public agencies, however, are normally eligible to enter the certification process as stock seed without routine certification agency site inspections. For contract grow-outs, however, this collection site information must be provided to the grower to enable certification.
Collection Timing
The USDI Bureau of Land Management, Seeds of Success crews made 29 pale agoseris seed collections from 2002 to 2016 in Oregon, California, Idaho, Nevada, Montana, Utah, Wyoming, and Colorado (USDI BLM SOS 2021). Most collections (16) were made in June. Harvests were also made in May (2), July (9), and September (2). The earliest collection date, May 25, 2012, was made at 3,491 ft (1,064 m) in elevation in Yellowstone County, Montana. The latest collection date, September 6, 2016, was made at 9,421 ft (2,872 m) in elevation in Gilpin County, Colorado. In the single year (2010) with the most collections (9), the earliest was June 7 at 6,658 ft (2,029 m) in elevation in Carbon County, Wyoming, and the latest was July 6 at 6,855 ft (2,089 m) in elevation in Tooele County, Utah. At many sites, multiple collections were made 3 to 8 days apart (USDI BLM SOS 2021).
In the Great Basin, seed is typically collected in June at 5,000 to 6,000 ft (1,500–1,800 m) in elevation (Jensen 2007). In this area, plants typically produce one or two seed heads annually, but with sufficient summer moisture additional flowering stalks may be produced. Seed matures uniformly over a 2-week period. First seed heads ripen about two weeks before subsequent seed head maturation. Since seed is naturally wind-dispersed, careful monitoring is important for timing wildland harvests. Seed maturation is signaled by the opening of the pappus (Jensen 2007).
Collection Methods
Plants often occur in low densities and must be hand collected (Fig. 9). Seed is stripped by gently grabbing the base of the seed head and pulling up with a loosely closed hand, focusing on collecting the seed rather than the stem or receptacle. This facilitates easier cleaning (Jensen 2007). Staff and researchers at the USDA Forest Service Provo Shrub Sciences Laboratory (PSSL) in Utah developed several tools and techniques for collecting small lots of pale agoseris (Jensen 2004). They built seed collection hoppers by sewing heavy-duty nylon cloth to a round frame such that the midpoint of the cloth dropped to form a basket to capture seeds. Hoppers also included adjustable straps to be worn around the collector’s neck and back to distribute the weight and bulk of the hopper. For plants with open seed heads, badminton racquets were used to sweep ripe seeds into the hopper. If conditions were windy, however, seeds were hand-plucked from the heads (Jensen 2004).
Figure 9. Collection of pale agoseris seed. Photo: USDI BLM WY030 SOS.
Several collection guidelines and methods should be followed to maximize the genetic diversity of wildland collections: 1) collect seed from a minimum of 50 randomly selected plants; 2) collect from widely separated individuals throughout a population without favoring the most robust or avoiding small stature plants; and 3) collect from all microsites including habitat edges (Basey et al. 2015). General collecting recommendations and guidelines are provided in online manuals (e.g., ENSCONET 2009; USDI BLM SOS 2021).
It is critical that wildland seed collection does not impact the sustainability of native plant populations. Collectors should take no more than 20% of the mature seed available at the time of harvest (USDI BLM SOS 2021). Additionally, care must be taken to avoid the inadvertent collection of weedy species, particularly those that produce seeds similar in shape and size to those of pale agoseris.
Post-Collection Management
Collections should be stored in breathable containers in cool, dry locations protected from insects and rodents. Because ripening pale agoseris seed commonly hosts developing insects, no-pest strips should be added to seed collections to prevent further damage (Jensen 2007).
Seed Cleaning
At the PSSL, staff cleaned small wildland seed lots by first screening to remove larger chaff. The remaining materials were rolled between two boards covered in soft leather until the pappus broke free from the seed. Final cleaning was done using air columns or an air screen machine (Jensen 2007).
Figure 10. Individual pale agoseris seeds with and without their pappus. Scale in mm. Photo: USDI BLM OR030 SOS.
Seed Storage
Pale agoseris seed is orthodox. No viability was lost after two months of storage at -4 °F (-20 °C) and 15% RH (SER, INSR, RBGK, SID 2023).
Seed Testing
There is no Association of Official Seed Analysts (AOSA) rule for testing germination or AOSA protocol for examining the viability of pale agoseris seed (AOSA 2010; 2016). Tetrazolium chloride testing (TZ) should follow that suggested for other Asteraceae species, which prescribes cutting seeds longitudinally leaving the distal end intact and soaking for 6 to 12 hours in 0.1% TZ concentration at (86–95 °F [30–35 °C]). The embryos of viable seed will be entirely stained red (AOSA 2010).
Belcher (1985) provided unofficial germination and viability tests. To test germination, seed is prechilled at 36 °F (2 °C) for 60 days then germinated at temperatures ranging from 53 to 63 °F (12–17 °C) or 63 to 72 °F (17–22 °C). Germination ranges from 40 to 90% and can be improved by soaking seed in 0.01 M solution of gibberellic acid (GA3) (Belcher 1985).
To test viability of pale agoseris seed, soak the seed in water for two hours, cut the radicle end, tease out the embryo, nick or scratch the inner seed coat, and place in 1% TZ to stain (Belcher 1985).
Germination Biology
A requirement for cold stratification of pale agoseris seed varies among seed lots. Karrfalt and Vankus (2009) reported that seed collected in the Great Basin germinated well without prechilling when exposed to constant temperatures of 41 to 68 °F (5–20 °C) and either 8 or 16 hours of light (Karrfalt and Vankus 2009). SER, INSR, RBGK, SID (2023) reported 100% germination after 7 days at 50 °F (10 °C) or 59 °F (15 °C) and 8 hours of light. For seed collected from a high-elevation site on the Dixie National Forest in southern Utah, germination with short-duration stratification (≤1 wk at 36 °F [2 °C]) was greater in cool temperatures (24%) than warm temperatures (0%). Highest germination (65%) was achieved with 8 weeks of dark stratification followed by incubation at 72/63 °F (22/17 °C) (McDonough 1970).
In greenhouse studies, pale agoseris germination was not affected by moderate or low levels of smoke exposure but was reduced by high levels (Cox 2016). Treatments included pale agoseris seed soaked with pure water, or high (1:10), moderate (1:100), or low (1:1000) smoke water concentrations for 20 hours. Seed was then put into germination boxes with two layers of germination paper, moistened to capacity. Germination was similar with no smoke, 100 and 1000 parts smoke but significantly lower with 10 parts smoke (Cox 2016).
Wildland Seed Yield And Quality
Post-cleaning seed yield and quality of seed lots collected in the Intermountain region are provided in Table 4 (USDA FS BSE 2017). The results indicate that pale agoseris seed lots can be cleaned to high levels of purity and seed fill and that purity of fresh seed is generally high.
Table 4. Seed yield and quality of pale agoseris seed lots collected in the Intermountain region, cleaned by the Bend Seed Extractory, and tested by the Oregon State Seed Laboratory or the USDA Forest Service National Seed Laboratory (USDA FS BSE 2017).
Seed lot characteristic | Mean | Range | Samples (no.) |
Bulk weight (lbs) | 0.61 | 0.03–4 | 32 |
Clean weight (lbs) | 0.14 | 0.004–1.8 | 32 |
Clean-out ratio | 0.19 | 0.015–0.62 | 32 |
Purity (%) | 96 | 90–99 | 32 |
Fill (%)¹ | 92 | 62–99 | 32 |
Viability (%)² | 92 | 85–97 | 12 |
Seeds/lb | 195,767 | 96,300–266,800 | 32 |
Pure live seeds/lb | 160,511 | 92,477–209,328 | 12 |
¹100 seed X-ray test
²Tetrazolium chloride test
Agricultural Seed Production
Studies of pale agoseris seed production in an agricultural setting were not available in the literature as of 2023.
Agricultural Seed Certification
In order to minimize genetic changes in specific accessions of native species when increased in cultivated fields, it is essential to track the geographic source and prevent inadvertent hybridization or selection pressure. This is accomplished by following third party seed certification protocols for Pre-Variety Germplasm (PVG) as established by the Association of Official Seed Certification Agencies (AOSCA). AOSCA members in the U.S., Canada, and other countries administer PVG requirements and standards that track the source and generation of planting stock. Field and cleaning facility inspections then monitor stand establishment, proper isolation distances, control of prohibited weeds, seed harvesting, cleaning, sampling, testing, and labeling for commercial sales (UCIA 2015; Young et al. 2020).
Seed growers apply for certification of their production fields prior to planting and plant only certified stock seed of an allowed generation (usually less than four). The systematic and sequential tracking through the certification process requires preplanning, knowing state regulations and deadlines, and is most smoothly navigated by working closely with state certification agency personnel. See the Wildland Seed Certification section for more information on stock seed sourcing.
Weed Management
Although herbicides are not approved for use in forb seed production, experimental studies by Roundy et al. (2005) found that pale agoseris seedling emergence was least impacted by imazapic treatments. Established seedlings were equally resistant to impazapic, pendimethalin, and imazethapyr herbicides at all rates (low = half the recommended rate, medium = the recommended rate, high = twice the recommended rate).
Pollinator Management
Jensen (2008) tried growing pale agoseris for seed production in a greenhouse. Plants grew poorly with only a few bolting. Lack of insect pollination precluded seed set.
The cavity-nesting Asteraceae specialist (Osmia californica) and the European honey bee (Apis mellifera) (Cane et al. 2012), can be transported to field locations in portable ground nests or hives. Providing these pollinators to seed production fields while encouraging and sustaining native bee populations may improve pale agoseris seed yields in fields (Cane 2008).
Pest Management
The following fungi utilize pale agoseris as a host plant but their potential to impact nursery or field growth is unknown: Bremia lactucae, Cladosporium agoseridis, Comoclathris compressa, Entyloma agoseridis, E. polysporum, Ovularia compacta, Puccinia columbiensis, P. crepidis-montanae, P. dioicae, P. extensicola, P. hieracii, P. patruelis, P. suksdorfii, P. troximontis, Ramularia agoseridis, R. compacta, Sphaerotheca humuli, and S. macularis (Farr and Rossman 2017). Fruit flies are also potential pests (Johnson 2008).
Nursery Practice
Jensen (2007) used the following procedure to grow pale agoseris plugs (7.5 cu in). Seed was wild collected at 7,200 ft (2,200 m) elevation in White Pine County, Nevada. Seeds were vertically planted in IHORT Q-plugs to the depth of the achene body. Trays were moistened, wrapped in plastic bags, and placed in a cold room for three weeks. Trays were then moved to a greenhouse (in January) and watered as needed. Greenhouse temperatures were 65 °F (18 °C) during the day and 50 °F (10 °C) at night. Germination occurred over a period of five weeks. At four weeks old, plants were fertilized biweekly using a liquid fertilizer (NPK 10-10-10). When nighttime temperatures increased to 65 °F (18 °C) two-month-old plants senesced within a week (Jensen 2007).
Wildland Seeding And Planting
Pale agoseris should be seeded 0 to 6.4 mm deep. Effects of seeding depth (0-1 in [2.5 cm]) on emergence and survival were evaluated in a greenhouse study. Pale agoseris seed was collected in June in Box Elder County, Utah, at 5,330 ft (1,615 m) elevation. Seed was planted in sandy loam (69% sand, 13% clay, 19% silt) and clay loam soils (35% sand, 30% clay, and 36% silt). Flats were kept moist in the greenhouse (81 °F [27 °C]). Emergence was best for seed sown on the soil surface and decreased with seeding depth. Emergence was better (though not significantly) in clay loam than sandy loam soils. Seeding depth did not have a significant effect on survival to 45 days but was better (though not significantly) in clay loam than sandy loam soils. Seed could likely be surface sown when water is not limiting or seeded to 6.4 mm in drier soils (Rawlins et al. 2009).
Pale agoseris established on amended and unamended mine spoils where it was seeded and where it was not purposely seeded (Smyth 1997). The three-year-old coal mine spoils were located at an alpine tundra and Engelmann spruce-subalpine fir transition zone in southeastern British Columbia. Vegetation establishment was evaluated on seeded and unseeded plots on unamended spoils and spoils amended with native soil. Unamended spoils were primarily siltstone and carbonaceous shale. Amended spoils were cleared of vegetation and covered with 2 in (5 cm) of native soil from an adjacent area, which was mixed with fertilizer (171 lbs/ac [192 kg/ha] N, 17 lbs/ac [19 kg/ha] each P and K). Seeded species were locally collected and stored at 36 to 41 °F (2–5 °C) until used. In germination tests for seeded species (68 °F [20 °C], 12 hr light and 12 hr dark), pale agoseris germination was 24%. Spoil plots were seeded in May 1985, and vegetation establishment was evaluated from 1986 to 1990. Pale agoseris was present with less than 1% of cover on all plots. Establishment on unseeded plots was attributed to seed dispersal of 3 to 7 ft (1–2 m) (Smyth 1997).
Acknowledgements
Funding for Western Forbs: Biology, Ecology, and Use in Restoration was provided by the USDI BLM Great Basin Native Plant Materials Ecoregional Program through the Great Basin Fire Science Exchange. Great thanks to the chapter reviewers: Robert Johnson, Brigham Young University, and Scott Jensen, USDA Forest Service, Rocky Mountain Research Station.
This research was supported in part by the USDA Forest Service, Rocky Mountain Research Station. The findings and conclusions in this publication are those of the authors and should not be construed to represent any official USDA or U.S. Government determination or policy.
Literature Cited
Aho, K.; Bala, J. 2012. Vascular alpine flora of Mount Washburn, Yellowstone National Park, USA. Madroño. 59(1): 2-13.
Aho, K.; Weaver, T. 2010. Alpine nodal ecology and ecosystem evolution in the north-central Rockies (Mount Washburn; Yellowstone National Park, Wyoming). Arctic, Antarctic, and Alpine Research. 42(2): 139-151.
Allen, E.A.; Nowak, R.S. 2008. Effect of pinyon-juniper tree cover on the soil seed bank. Rangeland Ecology and Management. 61(1): 63-73.
Andersen, B.A.; Holmgren, A.H. 1996. Mountain plants of northeastern Utah, revised. HG 506. Logan, UT: Utah State University Extension. 132 p.
Arno, S.F.; Habeck, J.R. 1972. Ecology of alpine larch (Larix lyallii Parl.) in the Pacific Northwest. Ecological Monographs. 42(4): 417-450.
Association of Official Seed Analysts [AOSA]. 2010. AOSA/SCST Tetrazolium testing handbook. Contribution No. 29. Lincoln, NE: Association of Official Seed Analysts.
Association of Official Seed Analysts [AOSA]. 2016. AOSA rules for testing seeds. Vol. 1. Principles and procedures. Washington, DC: Association of Official Seed Analysts.
Baird, G.I. 2006. Agoseris. In: Flora of North America Editorial Committee, ed. Flora of North America North of Mexico. Volume 19 Magnoliophyta: Asteridae (in part): Asteraceae, part 1. New York, NY: Oxford University Press: 323-334.
Baker, W.L. 1983. Alpine vegetation of Wheeler Peak, New Mexico, U.S.A.: Gradient analysis, classification, and biogeography. Arctic and Alpine Research. 15(2): 223240.
Barnett, J.K.; Crawford, J.A. 1994. Pre-laying nutrition of sage grouse hens in Oregon. Journal of Range Management. 47(2): 114-118.
Basey, A.C.; Fant, J.B.; Kramer, A.T. 2015. Producing native plant materials for restoration: 10 rules to collect and maintain genetic diversity. Native Plants Journal. 16(1): 37-53.
Bates, J.D.; Svejcar, T.J. 2009. Herbaceous succession after burning of cut western juniper trees. Western North American Naturalist. 69(1): 9-25.
Beatley, J.C. 1976. Vascular plants of the Nevada Test Site and central-southern Nevada: Ecologic and geographic distributions. Springfield, VA: U.S. Department of Commerce, Office of Technical Information, Technical Information Center. 308 p.
Belcher, E. 1985. Handbook on seeds of browse-shrubs and forbs. Tech. Pub. No. R8-TP8. Atlanta, GA: U.S. Department of Agriculture, Forest Service, Southern Region. 246 p.
Bentz, J.A.; Woodard, P.M. 1988. Vegetation characteristics and bighorn sheep use on burned and unburned areas in Alberta. Wildlife Society Bulletin. 16(2): 186193.
Blackwell, L.R. 2006. Great Basin wildflowers: A guide to common wildflowers of the high deserts of Nevada, Utah, and Oregon. Helena, MT: Morris Book Publishing. 288 p.
Boggs, C.L. 1988. Rates of nectar feeding in butterflies: Effects of sex, size, age and nectar concentration. Functional ecology. 2(3): 289295.
Bower, A.D.; St. Clair, J.B.; Erickson, V. 2014. Generalized provisional seed zones for native plants. Ecological Applications. 24(5): 913-919.
Bowns, J.E.; Bagley, C.F. 1986. Vegetation responses to long-term sheep grazing on mountain ranges. Journal of Range Management. 39(5): 431-434.
Branson, F.A.; Payne, G.F. 1958. Effects of sheep and gophers on meadows of the Bridger Mountains of Montana. Journal of Rangeland Management. 11(4): 165169.
Brazda, A.R. 1953. Elk migration patterns, and some of the factors affecting movements in the Gallatin River Drainage, Montana. The Journal of Wildlife Management. 17(1): 923.
Brink, V.C. 1964. Plant establishment in the high snowfall alpine and subalpine regions of British Columbia. Ecology. 45(3): 432438.
Burkle, L.; Irwin, R. 2009. The importance of interannual variation and bottom-up nitrogen enrichment for plant-pollinator networks. Oikos. 118(12): 1816-1829.
Cahill, J.F. 2003. Lack of relationship between below-ground competition and allocation to roots in 10 grassland species. Journal of Ecology. 91(4): 532540.
Cane, J.H. 2008. Pollinating bees crucial to farming wildflower seed for U.S. habitat restoration. In: James, R.; Pitts-Singer, T., eds. Bees in agricultural ecosystems. Oxford, UK: Oxford University Press: 48-64.
Cane, J.H.; Love, B.; Swoboda, K. 2012. Breeding biology and bee guild of Douglas’ dustymaiden, Chaenactis douglasii (Asteraceae, Helenieae). Western North American Naturalist. 72(4): 563-568.
Chambers, J.C. 1995. Relationships between seed fates and seedling establishment in an alpine ecosystem. Ecology. 76(7): 21242133.
Consortium of Pacific Northwest Herbaria [CPNWH]. 2020. Seattle, WA: University of Washington Herbarium, Burke Museum of Natural History and Culture. http://www.pnwherbaria.org/index.php
Cook, C.W. 1983. “Forbs” need proper ecological recognition. Rangelands. 5(5): 217-220.
Cox, R. 2016. Smoke-induced germination of Great Basin native forbs. In: Kilkenny, F; Edwards, F.; Malcomb, A., eds. Great Basin Native Plant Project: 2015 Progress Report. Boise, ID: U.S. Department of Agriculture, Forest Service, Rocky Mountain Research Station: 78-82.
Craighead, J.J.; Craighead, F.C. Jr.; Davis, R.J. 1963. A field guide to Rocky Mountain wildflowers from northern Arizona and New Mexico to British Columbia. Boston, MA: Houghton Mifflin Company. 277 p.
Cronquist, A. 1994. Volume five: Asterales. In: Cronquist, A.; Holmgren, A.H.; Holmgren, N.H.; Reveal, J.L.; Holmgren, P.K., eds. Intermountain flora: Vascular plants of the Intermountain West, U.S.A. Bronx, NY: The New York Botanic Garden. 496 p.
Currah, R.; Smreciu, A.; Van Dyk, M. 1983. Prairie wildflowers: An illustrated manual of species suitable for cultivation and grassland restoration. Edmonton, AB: University of Alberta, Friends of the Devonian Botanic Garden. 290 p.
Despain, D.G. 1973. Vegetation of the Big Horn Mountains, Wyoming, in relation to substrate and climate. Ecological Monographs. 43(3): 329355.
Douglas, G.W.; Bliss, L.C. 1977. Alpine and high subalpine plant communities of the North Cascades Range, Washington and British Columbia. Ecological Monographs. 47(2): 113-150.
Ehleringer, J.R.; Arnow, L.A.; Arnow, T.; McNulty, I.B.; McNulty, I.R.; Negus, N.C. 1992. Red Butte Canyon Research Natural Area: History, flora, geology, climate, and ecology. The Great Basin Naturalist. 52(2): 95121.
Eldredge, E.; Novak-Echenique, P.; Heater, T.; Mulder, A.; Jasmine, J. 2013. Plants for pollinator habitat in Nevada. Tech. Note NV 57. Reno, NV: U.S. Department of Agriculture, Natural Resources Conservation Service. 65 p.
Eshelman, B.D.; Jenkins, S.H. 1989. Food selection by Belding’s ground squirrels in relation to plant nutritional features. Journal of Mammalogy. 70(4): 846852.
European Native Seed Conservation Network [ENSCONET]. 2009. ENSCONET seed collecting manual for wild species. Edition 1: 32 p.
Evenson, W.E.; Brotherson, J.D.; Wilcox, R.B. 1980. Relationship between environmental and vegetational parameters for understory and open-area communities. The Great Basin Naturalist. 40(2): 167174.
Ezzeddine, M.; Matter, S.F. 2008. Nectar use and electivity by butterflies in sub-alpine meadows. Journal of the Lepidopterists Society. 62(3): 138142.
Farr, D.F.; Rossman, A.Y. 2017. Fungal databases, U.S. National Fungus Collections. U.S. Department of Agriculture, Agricultural Research Service. https://nt.ars-grin.gov/fungaldatabases/.
Feuer, S.; Tomb, A.S. 1977. Pollen morphology and detailed structure of family Compositae, tribe Cichorieae. II. subtribe Microseridinae. American Journal of Botany. 64(2): 230-245.
Hansen, R.M.; Ueckert, D.N. 1970. Dietary similarity of some primary consumers. Ecology. 51(4): 640-648.
Hart, E.B. 1992. Tamias dorsalis. Mammalian Species. 399: 16.
Hersperger, A.M.; Forman, R.T.T. 2003. Adjacency arrangement effects on plant diversity and composition in woodland patches. Oikos. 101(2): 279-290.
Hickman, J.C., ed. 1993. The Jepson manual: Higher plants of California. Berkeley, CA: University of California Press. 1400 p.
Hitchcock, C.L.; Cronquist, A. 2018. Flora of the Pacific Northwest: An illustrated manual. Second Ed. Giblin, D.E.; Legler, B.S.; Zika, P.F.; Olmstead, R.G., eds. Seattle, WA: University of Washington Press. 882 p.
Hungerford, C.R. 1970. Response of Kaibab mule deer to management of summer range. The Journal of Wildlife Management. 34(4): 852862.
Hurd, R.M. 1961. Grassland vegetation in the Big Horn Mountains, Wyoming. Ecology. 42(3): 459467.
Hurd, R.M.; Pond, F.W. 1958. Relative preference and productivity of species on summer cattle ranges, Big Horn Mountains, Wyoming. Journal of Range Management. 11(3): 109114.
Jenkins, S.H.; Bollinger, P.W. 1989. An experimental test of diet selection by the pocket gopher Thomomys monticola. Journal of Mammalogy. 70(2): 406412.
Jensen, M.E.; Peck, L.S.; Wilson, M.V. 1988. Vegetation characteristics of mountainous northeastern Nevada sagebrush community types. The Great Basin Naturalist. 48(4): 403-421.
Jensen, S. 2004. Racquets, hoppers, and felt boards – Low-tech devices for processing seeds. Native Plants Journal. 5(1): 50-51.
Jensen, S. 2005. Toward selected releases of Agoseris, Astragalus, Lupinus, Phlox, Tragopogon, and Stipa. In: Shaw, N.; Pellant, M. 2004. Great Basin Native Plant Selection and Increase Project: 2004 Annual Report. Boise, ID: U.S. Department of Agriculture, Rocky Mountain Research Station: 14.
Jensen, S. 2007. Propagation protocol for production of container (7.5 cu in Qplugs) Agoseris glauca (Pursh) Raf. seeds. Native Plant Network. U.S. Department of Agriculture, Forest Service, National Center for Reforestation, Nurseries, and Genetic Resources. http://npn.rngr.net/propagation/protocols [Accessed 2022 August 09].
Johnson, R.L. 2008. Impacts of habitat alterations and predispersal seed predation on the reproductive success of Great Basin forbs. Provo, UT: Brigham Young University. Dissertation. 102 p.
Knight, R.R. 1970. The Sun River elk herd. Wildlife Monographs. 23: 366.
Kufeld, R.C. 1973. Foods eaten by the Rocky Mountain elk. Journal of Range Management. 26(2): 106-113.
LaBerge, W.E. 1980. A revision of the bees of the genus Andrena of the Western Hemisphere. Part X. subgenus Andrena. American Entomological Society. 106(4): 395525.
LaBerge, W.E. 1989. A revision of the bees of the genus Andrena of the Western Hemisphere. Part XIII. Subgenera Simandrena and Taeniandrena. Transactions of the American Entomological Society. 115(1): 1-56.
Laberge, W.E.; Ribble, D.W. 1975. A revision of the bees of the genus Andrena of the Western Hemisphere. Part VII. Subgenus Euandrena. Transactions of the American Entomological Society. 101(3): 371-446.
Lady Bird Johnson Wildflower Center [LBJWC]. 2009. Agoseris glauca (Pursh) Raf. Native Plant Database. Austin, TX: Lady Bird Johnson Wildflower Center. https://www.wildflower.org/plants-main [Accessed 2022 August 9].
Larson, J.; Reif, B.; Nelson, B.E.; Hartman, R.L. 2014. Floristic studies in north central New Mexico, U.S.A. The Sangre de Cristo Mountains. Journal of the Botanical Research Institute of Texas. 8(1): 271-303.
Laughlin, D.C.; Bakker, J.D.; Fulé; P.Z. 2005. Understorey plant community structure in lower montane and subalpine forests, Grand Canyon National Park, USA. Journal of Biogeography. 32(12): 2083-2102.
Lavin, M. 1983. Floristics of the upper Walker River, California and Nevada. The Great Basin Naturalist. 43(1): 93-130.
Laycock, W.A. 1967. How heavy grazing and protection affect sagebrush-grass ranges. Journal of Range Management. 20(4): 206-213.
Lesica, P. 2012. Manual of Montana vascular plants. Fort Worth, TX: Botanical Research Institute of Texas (BRIT) Press. 779 p.
Looman, J. 1969. The fescue grasslands of western Canada. Vegetatio. 19(1/6): 128-145.
Luna, T.; Mousseaux, M.R.; Dumroese, R.K. 2018. Common native forbs of the northern Great Basin important for greater sage-grouse. Gen. Tech. Rep. RMRS-GTR-387. Fort Collins, CO: U.S. Department of Agriculture, Forest Service, Rocky Mountain Research Station; Portland, OR: U.S. Department of the Interior, Bureau of Land Management, Oregon-Washington Region. 76 p.
Mansfield, D.H. 2000. Flora of Steens Mountain. Corvallis, OR: Oregon State University Press. 410 p.
Martinsen, D.L. 1968. Temporal patterns in the home ranges of chipmunks (Eutamias). Journal of Mammalogy. 49(1): 8391.
McDonough, W.T. 1970. Germination of 21 species collected from a high-elevation rangeland in Utah. The American Midland Naturalist. 84(2): 551554.
McInnis, M.L.; Vavra, M.; Krueger, W.C. 1983. A comparison of four methods used to determine the diets of large herbivores. Journal of Range Management. 36(3): 302-306.
Moerman, D. 2003. Native American ethnobotany: A database of foods, drugs, dyes, and fibers of Native American peoples, derived from plants. Dearborn, MI: University of Michigan. http://naeb.brit.org
Nelle, P.J.; Reese, K.P.; Connelly, J.W. 2000. Long-term effects of fire on sage grouse habitat. Journal of Range Management. 53(6): 586-591.
Ogle, D.; Brazee, B. 2009. Estimating initial stocking rates. Range Tech. Note 3. Boise, ID: U.S. Department of Agriculture, Natural Resources Conservation Service. 11 p.
Omernik, J.M. 1987. Ecoregions of the conterminous United States. Map (scale 1:7,500,000). Annals of the Association of American Geographers. 77(1): 118-125.
Ostler, W.K.; Harper, K.T.; McKnight, K.B.; Anderson, D.C. 1982. The effects of increasing snowpack on a subalpine meadow in the Uinta Mountains, Utah, U.S.A. Arctic and Alpine Research. 14(3): 203214.
Pennington, V.E.; Schlaepfer, D.R.; Beck, J.L.; Bradford, J.B.; Palmquist, K.A.; Lauenroth, W.K. 2016. Sagebrush, greater sage-grouse, and the occurrence and importance of forbs. Western North American Naturalist. 76(3): 298-312.
Plant Conservation Alliance [PCA]. 2015. National seed strategy for rehabilitation and restoration 2015-2020. Washington, DC: U.S. Department of the Interior, Bureau of Land Management. 52 p.
Poreda, S.F.; Wullstein, L.H. 1994. Vegetation recovery following fire in an oakbrush vegetation mosaic. Great Basin Naturalist. 54(4): 380-383.
Rawlins, J.K.; Anderson, V.J.; Johnson, R.; Krebs, T. 2009. Optimal seeding depth of five forb species from the Great Basin. Native Plants Journal. 10(1): 33-42.
Reed, J.F. 1952. The vegetation of the Jackson Hole Wildlife Park, Wyoming. The American Midland Naturalist. 48(3): 700-729.
Reed, R.M. 1976. Coniferous forest habitat types of the Wind River Mountains, Wyoming. The American Midland Naturalist. 95(1): 159173.
Richardson, B.; Kilkenny, F.; St. Clair, B.; Stevenson-Molnar, N. 2020. Climate Smart Restoration Tool. https://climaterestorationtool.org/csrt/
Richardson, C.A.; Henderson, D.M. 1976. Classification and ordination of the alpine plant communities of Railroad Ridge, White Cloud Peaks, Idaho. The Great Basin Naturalist. 59(1): 6378.
Roundy, B.; Anderson, V.J.; Johnson, R. 2005. Forb production cultural practices. In: Shaw, N.; Pellant, M., eds. Great Basin Native Plant Project: 2004 Progress Report. Boise, ID: U.S. Department of Agriculture, Forest Service, Rocky Mountain Research Station: 38-42.
Sampson, A.W. 1917. Important range plants: Their life history and forage value. Bulletin 545. Washington, D.C.: U.S. Department of Agriculture. 63 p.
Schiebout, M.H.; Hazlett, D.L.; Snow, N. 2008. A floristic survey of vascular plants over parts of northeastern New Mexico. Journal of the Botanical Research Institute of Texas. 2(2): 14071447.
SEINet – Regional Networks of North American Herbaria Steering Committee [SEINet]. 2020. SEINet Regional Networks of North American Herbaria. https://Symbiota.org/docs/seinet
Smith, A.D. 1953. Consumption of native forage species by captive mule deer during summer. Journal of Range Management. 6(1): 30-37.
Smith, D.R. 1967. Gross energy value of aboveground parts of alpine plants. Journal of Range Management. 20(3): 179180.
Smyth, C.R. 1997. Early succession patterns with a native species seed mix on amended and unamended coal mine spoil in the Rocky Mountains of southeastern British Columbia, Canada. Arctic and Alpine Research. 29(2): 184-195.
Society for Ecological Restoration; International Network for Seed Based Restoration; Royal Botanic Gardens [SER, INSR, RBGK]. 2023. Seed Information Database (SID). Available from: https://ser-sid.org/
Spellenberg, R. 2001. National Audubon Society field guide to North American wildflowers: Western region, revised edition. New York, NY: Alfred A. Knopf, Inc. 862 p.
Stevens, D.R. 1966. Range relationships of elk and livestock, Crow Creek Drainage, Montana. Journal of Wildlife Management. 30(2): 349-363.
Stevens, O.A. 1957. Weights of seeds and numbers per plant. Weeds. 5(1): 46-55.
Stubbendieck, J.; Hatch, S.L.; Hirsch, K.J. 1986. North American range plants. 3rd ed. Lincoln, NE: University of Nebraska Press. 465 p.
Taylor, R.J. 1992. Sagebrush country: A wildflower sanctuary. Missoula, MT: Mountain Press Publishing Company. 211 p.
Thomson, J.D. 1981. Field measures of flower constancy in bumblebees. The American Midland Naturalist. 105(2): 377380.
Titus, J.H.; Moore, S.; Arnot, M.; Titus, P.J. 1998. Inventory of the vascular flora of the blast zone, Mount St. Helens, Washington. Madroño. 45(2): 146-161.
Tueller, P.T.; Eckert, R.E. 1987. Big sagebrush (Artemisia tridentata vaseyana) and longleaf snowberry (Symphoricarpos oreophilus) plant associations in northeastern Nevada. The Great Basin Naturalist. 47(1): 117-131.
Turner, N.J.; Thompson, L.C.; Thompson, M.T.; York, A.Z. 1990. Thompson ethnobotany: Knowledge and usage of plants by the Thompson Indians of British Columbia. Royal British Columbia Museum Memoir No. 3. Victoria, British Columbia, Canada: Royal British Columbia Museum. 335 p.
U.S. Department of Agriculture, Forest Service [USFS]. 1937. Range Plant Handbook. Washington, DC: U.S. Department of Agriculture, Forest Service. 816 p.
USDA Forest Service, Bend Seed Extractory [USDA FS BSE]. 2017. Nursery Management Information System Version 4.1.11. Local Source Report 34-Source Received. Bend, OR: U.S. Department of Agriculture, Forest Service, Bend Seed Extractory.
USDA Forest Service, Western Wildland Environmental Threat Assessment Center [USFS WWETAC]. 2017. TRM Seed Zone Applications. Prineville, OR: U.S. Department of Agriculture, Forest Service, Western Wildland Environmental Threat Assessment Center. https://www.fs.fed.us/wwetac/threat-map/TRMSeedZoneMapper.php
USDA Natural Resources Conservation Service [USDA NRCS]. 2022. The PLANTS Database. Greensboro, NC: U.S. Department of Agriculture, Natural Resources Conservation Service, National Plant Data Team. https://plants.usda.gov/java
USDI Bureau of Land Management, Seeds of Success [USDI BLM SOS]. 2017. Seeds of Success collection data. Washington, DC: U.S. Department of the Interior, Bureau of Land Management, Plant Conservation and Restoration Program.
USDI Bureau of Land Management, Seeds of Success [USDI BLM SOS]. 2021. Bureau of Land Management technical protocol for the collection, study, and conservation of seeds from native plant species for Seeds of Success. Washington, DC: U.S. Department of the Interior, Bureau of Land Management, Plant Conservation and Restoration Program. 44 p.
USDI Environmental Protection Agency [USDI EPA]. 2017. Ecoregions. Washington, DC: U.S. Department of the Interior, Environmental Protection Agency. https://www.epa.gov/eco-research/ecoregions
USDI Geological Survey [USDI USGS]. 2020. Biodiversity Information Serving Our Nation (BISON). U.S. Geological Survey. https://www.gbif.us/#home
Utah Crop Improvement Association [UCIA]. 2015. How to be a seed connoisseur. Logan, UT: UCIA, Utah Department of Agriculture and Food, Utah State University and Utah State Seed Laboratory. 16 p.
Utah State University Extension [USU Ext]. 2017. Range plants of Utah: Mountain dandelion. Logan, UT: Utah State University. https://extension.usu.edu/rangeplants/forbs-herbaceous/mountain-dandelion [Accessed 2022 December 3].
Vaughan, T.A. 1974. Resource allocation in some sympatric, subalpine rodents. Journal of Mammalogy. 55(4): 764-795.
Vaughan, T.A.; Weil, W.P. 1980. The importance of arthropods in the diet of Zapus princeps in a subalpine habitat. Journal of Mammalogy. 61(1): 122124.
Watt, W.B.; Hoch, P.C.; Mills, S.G. 1974. Nectar resource use by Colias butterflies. Chemical and visual aspects. Oecologia. 14(4): 353-374.
Weaver, T. 1974. Ecological effects of weather modification: Effect of late snowmelt on Festuca idahoensis Elmer Meadows. The American Midland Naturalist. 92(2): 346356.
Welsh, S.L.; Atwood, N.D.; Goodrich, S.; Higgins, L.C., eds. 2015. A Utah Flora. Fifth Edition, revised. Provo, UT: Brigham Young University. 990 p.
Wilkins, B.T. 1957. Range use, food habits, and agricultural relationships of the mule deer, Bridger Mountains, Montana. The Journal of Wildlife Management. 21(2): 159-169.
Wilson, J.S.; Wilson, L.E.; Loftis, L.D.; Griswold, T. 2010. The montane bee fauna of north central Washington, USA, with floral associations. Western North American Naturalist. 70(2): 198-207.
Wynd, F.L. 1936. The flora of Crater Lake National Park. The American Midland Naturalist. 17(6): 881949.
Young, S.A.; Schrumpf, B.; Amberson, E. 2003. The Association of Official Seed Certifying Agencies (AOSCA) native plant connection. Moline, IL: AOSCA. 9 p. Available: https://seedcert.oregonstate.edu/sites/seedcert.oregonstate.edu/files/pdfs/aoscanativeplantbrochure.pdf
How to Cite
Gucker, C.L.; Shaw, N.L. 2023. Pale agoseris (Agoseris glauca [Pursh] Raf.). In: Gucker, C.L.; Shaw, N.L., eds. Western forbs: Biology, ecology, and use in restoration. Reno, NV: Great Basin Fire Science Exchange. https://westernforbs.org/species/pale-agoseris-agoseris-glauca/