Authorship
Gucker, Corey; Shaw, Nancy
Publication Date
August 2020
Nomenclature
Gray’s biscuitroot (Lomatium grayi [J.M. Coult. & Rose] J.M. Coult. & Rose) belongs to the Apiaceae family and Apioideae subfamily.
Family
Apiaceae – Carrot family
Genus
Lomatium
Species
grayi
NRCS Plant Code
LOGR (USDA NRCS 2019).
Subtaxa
Lomatium grayi var. depauperatum, L. t. var. grayi (USDA NRCS 2019).
Synonyms
Cogswellia grayi (Coulter and Rose 1909).
Common Names
Gray’s biscuitroot, Gray’s desert-parsley, Gray’s lomatium, foothill biscuitroot, Indian celery, milfoil lomatium, mountain desert-parsley (Williams and Ralphs 1989; Norton et al. 1999; Sun and Downie 2010; USU Ext. 2017).
Chromosome Number
Chromosome number is: 2n = 22 (Rice et al. 2015).
Hybridization
No hybridization has been documented for Lomatium species (Hitchcock and Cronquist 2018).
Distribution
Gray’s biscuitroot is one of the most widespread Lomatium species (Thompson 1987a). It is most common in the western US from eastern Washington and Oregon south to northeastern Nevada, western Wyoming, western Colorado, and northwestern New Mexico (Coulter and Rose 1900; Hermann 1966). Populations occur from near sea level to near alpine habitats. Populations on the Saltspring and Galiano islands of British Columbia represent disjunct populations that are the northernmost and coastalmost. Populations in California, like those in British Columbia, are rare and disjunct (COSEWIC 2008). Variety grayi occurs throughout the species range, and variety depauperatum occurs only in Nevada and Utah (Kartesz 2015).
Habitat And Plant Associations
Gray’s biscuitroot has a wide ecological range. It commonly occurs in rocky, dry to moist soils on cliffs, outcrops, slopes, and in draws at low to mid-elevations (Taylor 1992; Andersen and Holmgren 1996; Pavek et al. 2012; USU Ext. 2017; Hitchcock and Cronquist 2018). It occurs in grasslands (Fig. 1), sagebrush (Artemisia spp.) (Fig. 2), and openings in mountain shrub, pinyon-juniper (Pinus–Juniperus spp.), ponderosa pine (P. ponderosa), and Douglas-fir (Pseudotsuga menziesii) communities at sites receiving 8 to 20 in (200–500 mm) of annual precipitation (Tilley et al. 2011). Gray’s biscuitroot is often associated with bluebunch wheatgrass (Pseudoroegneria spicata), bluegrasses (Poa spp.), arrowleaf balsamroot (Balsamorhiza sagittata), and lupines (Lupinus spp.) (USDA FS 1937; Thompson 1987b).
Figure 1. Gray’s biscuitroot growing with grasses at a rocky soil site in Oregon. Photo: USDI BLM OR931 SOS.
Figure 2. Gray’s biscuitroot growing in a draw in a sagebrush community, a typical habitat. Photo: USFS.
In many locations, Gray’s biscuitroot grows in dry, rocky habitats. On the Gulf Islands of British Columbia, it occurs on very steep, south-facing, rock walls (Fig. 3) in small pockets of soil (COSEWIC 2008). In eastern Washington, it grows on south-facing banks of the Spokane River, where insolation was intense, winds created exceedingly dry conditions, and vegetation (smooth sumac [Rhus glabra], buckwheat [Eriogonum spp.], and lupine) are widely spaced (Turesson 1914). On the Snake River Plain in southeastern Idaho, it occurs in isolated patches of big sagebrush (Artemisia tridentata) within a matrix of relatively barren volcanic basalt (Huntly et al. 2011). In other parts of the west, Gray’s biscuitroot occurs in Oregon white oak (Quercus garryana)-dominated forests (Wolter and Fonda 2002), scabland sagebrush (A. rigida)-bluegrass and thymeleaf buckwheat (E. thymoides)-bluegrass communities (Poston 1995), little sagebrush (A. arbuscula)/bluebunch wheatgrass vegetation, rigid sagebrush scablands (Hall 2007), and cold desert communities with shadscale saltbush (Atriplex confertifolia) and rubber rabbitbrush (Ericameria nauseosa) (Bolen 1964).
Figure 3. Gray’s biscuitroot plants growing in a rock wall in Oregon. Photo: BLM OR030 SOS.
Elevation
Gray’s biscuitroot occurs from near sea level to subalpine elevations but is most common at mid-elevation sites below 6,890 ft (2,100 m) (Hitchcock and Cronquist 2018).
Soils
Gray’s biscuitroot grows in a wide variety of soil types across its range, but soils are often rocky, shallow, and well-drained (Thompson 1984; COSEWIC 2008; USU Ext. 2017). In coastal British Columbia, most Gray’s biscuitroot populations grew in soils of Cretaceous conglomerates and sandstones, although some grew in older metamorphic rock (Fig. 3) (COSEWIC 2008). In eastern Washington’s Adams County, Gray’s biscuitroot occurred in scablands where soils were 50% sand, 40% silt, and 10% clay and had a pH of 5 to 8 (Crowe et al. 1994). In the Columbia Basin and other eastern Washington and Oregon locations, Gray’s biscuitroot dominated herbaceous communities in ephemeral and intermittent streambeds (Fig. 2) with sandy loam or sandy clay loam soils and 58% gravel (Crawford 2003). In the Green Canyon area of Cache County, Utah, Gray’s biscuitroot occurred in hard, rocky soils with little humus and pH of 8.1. Soils were silt loams with 50% silt, 37% fine sand, and 13% clay (Nye 1980). In northwestern Colorado’s Elkhead Watershed, Gray’s biscuitroot occurred on clay pan sites with thin clay or clay loam A horizons and fine-textured subsoils that restrict water movement and availability (Kachergis et al. 2012).
Soil conditions may impact Gray’s biscuitroot survival. Small mammals commonly feed on the roots of Gray’s biscuitroot. These predators killed far fewer plants at sites where plants grow in shallow soils in fissures between basaltic outcroppings than plants growing in shallow, rocky soils (Thompson 1987b).
Description
Gray’s biscuitroot is a large, bushy, perennial with a woody taproot (Fig. 4) (Pavek et al. 2012; Hitchcock and Cronquist 2018). Plants can reach 3 ft (0.9 m) tall and 2 ft (0.6 m) wide with roots extending 5 or more ft (1.5 m) deep (Weaver 1915; USU Ext. 2017). Gray’s biscuitroot excavated from prairie habitats in southeastern Washington had long, fleshy, poorly branched taproots that were flattened, folded, and twisted as they grew through narrow crevices between rocks to depths of 3 ft (0.9 m) or more (Weaver 1915).
Figure 4. Young Gray’s biscuitroot plant, crown, and taproot. Photo: USDA USFS.
Plants are acaulescent or short caulescent with leaves and flower stalks arising from the branching crown of a thick taproot (Fig. 4) (Mathias 1938; Hermann 1966; USU Ext. 2017). Herbage is glabrous to scabrous with a strong, parsley-like odor when crushed (Mathias 1938; Taylor 1992). Leaves are basal (Craighead et al. 1963) and pinnately divided three times into numerous fernlike segments that turn in different planes, which at first glance makes them appear thicker than they actually are (Pavek et al. 2012; USU Ext. 2017). Leaves have 1.4 to 8.7 in (3.5–22 cm) long petioles and broadly obovate blades 4 to 10 in (10.5–25 cm) long with very fine, hairlike, terminal leaflets (1–3 mm by 0.2–0.6 mm) (Fig. 5) (Mathias 1938; Craighead et al. 1963; Hitchcock and Cronquist 2018).
Figure 5. Gray’s biscuitroot growing on rocky soil in Owyhee County, Idaho. Photo: USDA USFS.
Mature plants produce male and bisexual flowers in large, flat-topped compound umbels (Fig. 6) (Thompson 1984). Umbels arise independently from the root crown on slender flower stalks up to 2 ft (0.6 m) tall (Craighead et al. 1963; Thompson 1984; COSEWIC 2008; Pavek et al. 2012; USU Ext. 2017). Stems (mean 2.3 mm diameter) of umbels producing only male flowers are significantly smaller than stems (mean 3.5 mm diameter) of umbels with some bisexual flowers based on measurements from plants growing near Clarkston, Washington (P < 0.01). Stems supporting all male flowers wither quickly while stems with bisexual flowers remain green and erect much longer (Thompson 1983). Umbels have 7 to 22 rays, which spread 0.8 to 6 in (2–15 cm) and support umbellets with 15 to 30 flowers (Coulter and Rose 1900; Mathias 1938). Bases of umbellets are encircled with small, narrow bracts (USDA FS 1937).
Bisexual flowers are small with five bright yellow petals, five stamens, and no sepals (Taylor 1992). Single pistils are bicarpellate with an inferior ovary, two styles, and oily endosperm. Fruits (schizocarps) split into two dry, single-seeded mericarps at maturity (USDA FS 1937; Taylor 1992). Fruit and seed size are variable. Schizocarps are flattened dorsally, oblong, 0.3 to 0.6 in (6.5–16 mm) long and 0.2 to 0.3 in (4–9 mm) wide with winged edges that are 15 to 70% as wide as the fruit body (Coulter and Rose 1900; Mathias 1938; Hitchcock and Cronquist 2018). Fruit and seed size are variable. In an experimental garden, Gray’s biscuitroot seed mass differed by 15.8 times for 2-year-old plants grown under similar conditions and up to 8.1 times within individual plants (Thompson 1984). Gray’s biscuitroot produces up to 20 or more umbels each of which contains several hundred male and bisexual flowers (Thompson 1984).
Figure 6. Compound bisexual umbel of Gray’s biscuitroot in flower with a few fruits beginning to develop. Photo: USDA USFS.
Reproduction
Flowering and seed production of Gray’s biscuitroot generally begins when plants are 2 or 3 years old and is highly variable (i.e. may not occur every year) (Thompson and Moody 1985). Flowering and seed production were monitored for Gray’s biscuitroot plants grown from seeds collected in eastern Washington. Plants were grown for 3 years, first in the greenhouse and then outdoors. Only 2 of 13 plants that flowered in the first year produced bisexual flowers and none of those set seed. Eighteen of the 21 plants that flowered in the second year produced at least one umbel with some bisexual flowers, and all 18 produced seed. On average of 65% of umbels produced bisexual flowers (range: 0–100%), and 68% of bisexual umbels produced seed (Thompson 1984).
Common garden and population studies revealed that flowering, seed production, and life history of Gray’s biscuitroot vary by population. In the common garden near Washington State University, where steppe vegetation would dominate, plants grown from seed collected from meadow steppe vegetation on Smoot Hill (18 km north of the common garden) grew faster, flowered earlier, flowered at smaller sizes, and produced more flowers than plants grown from seed collected from grasslands of the Blue Mountains near Clarkston, Washington (about 26 mi (45 km)south of the Smoot Hill population (Thompson and Moody 1985; Thompson 1987b). For both populations, the probability of flowering depended upon plant size and was greater for large plants than small plants (P < 0.0001). The percentage of bisexual flowers per plant increased with plant age and was consistently higher for Clarkston than Smoot Hill plants (P < 0.0001). Over the 6-year study, bisexual flowers producing mature schizocarps varied from 50 to 70% for Clarkston plants and 35 to 63% for Smoot Hill plants. Smoot Hill plants were adapted for rapid growth and early reproduction (Thompson and Moody 1985), and at the Smoot Hill site, plants had relatively short lifetimes. Most deaths were caused by burrowing and grazing mammals (Thompson 1987b).
Phenology
Gray’s biscuitroot typically produces flowers in spring (April-June) (Ogle et al. 2019).
Pollination
Bees and flies were observed pollinating Gray’s biscuitroot flowers in Utah (Nye 1980; Cane 2004). Sweat bees visited Gray’s biscuitroot for nectar and pollen in April and May. In Green Canyon, Cache County, Utah, the first overwintering female sweat bees (Halictus farinosus) emerged on April 1 and continued emerging until early May. On June 9, 60% of a pollen ball collected from a solitary sweat bee nest was from Gray’s biscuitroot (Nye 1980).
Ecology
Gray’s biscuitroot is a moderately long-lived (≥7 yrs), perennial that completes its annual life cycle early in the growing season (Thompson 1987b; Skinner et al. 2005; Ogle et al. 2012). Growth begins extremely early. Plants green up soon after snowmelt, and seed matures by early summer (USDA FS 1937; Tilley et al. 2011). Plants produce a large taproot, which suggests they could survive aboveground disturbances. However, plants are susceptible to below-ground feeding by small mammals (Thompson 1987b).
Seed And Seedling Ecology
An in-depth study of four large Gray’s biscuitroot populations in southeastern Washington found that the initial size structure of seedling populations was partly dependent on the direct and indirect effects of seed parasites (Ellison and Thompson 1987). Selected populations, two in bluebunch wheatgrass and two in subalpine meadows, all supported one or more insect parasites. The frequency of umbels containing snout beetle weevils (Smicronyx spp.) varied from 8 to 33% over the season, and the number of adults per umbel varied 1 to 15. Larval feeding killed most of the attacked seeds, and very few larvae-damaged seeds germinated (6.1%). Plant mass was 50 to 75% lower for the few seedlings grown from larval-damaged seed than for control seeds. With any added stress such as reduced light or growing with competition from cheatgrass (Bromus tectorum), the influence of seed size on Gray’s biscuitroot plant mass in the first year of growth became important. Under competitive conditions, 24 to 31% of the variance in plant mass was explained by seed mass. Researchers noted that because Gray’s biscuitroot seedlings experience highly variable growing seasons year to year, seed mass to seedling and plant mass correlations may not always be a significant factor in this species (Ellison and Thompson 1987).
Disturbance Ecology
Apart from studies on herbivory and granivory (see Wildlife and Livestock Use, Seed and Seedling Ecology), few studies have directly evaluated the susceptibility of Gray’s biscuitroot to disturbances or top kill.
Wildlife And Livestock Use
Gray’s biscuitroot provides good spring forage for large and small mammals, greater sage-grouse (Centrocercus urophasianus), and many insects (Ogle et al. 2019). Heavy herbivory is considered a reason that many populations are small, isolated, and restricted to sites with difficult access (COSEWIC 2008; Tilley et al. 2011).
Mammals. Elk (Cervus canadensis), deer (Odocoileus spp.), pronghorn (Antilocapra americana), and livestock feed on biscuitroot (Lomatium spp.) (USDA FS 1937; Hermann 1966; Ogle and Brazee 2009). Domestic sheep eat all parts of the plant including green seeds (USDA FS 1937). In a cultivated field, Gray’s biscuitroot was eaten by rodents and eastern cottontails (Sylvilagus floridanus) (COSEWIC 2008). When diets of Idaho ground squirrels (Urocitellus brunneus) and Columbian ground squirrels (U. columbianus) were evaluated at two sites in Adams County, Idaho, biscuitroot species were found to be important to both species. At one site, Gray’s biscuitroot was the second most important species in Idaho ground squirrel diets (Dyni and Yensen 1996).
In the coastal areas of British Columbia, Gray’s biscuitroot populations are considered isolated and small because of herbivory (COSEWIC 2008). At one site, about 60 small seedlings were found crowded on a flat, easily accessible surface about 6.6 ft (2 m) beneath a large, mature plant growing out of a vertical rock wall. The lack of large plants on the accessible substrate was attributed to deer and sheep grazing. This theory was further supported when cultivated specimens were eaten by rodents and rabbits. Gray’s biscuitroot’s strong parsnip-like smell was thought to attract small mammals (COSEWIC 2008).
Birds. Gray’s biscuitroot and other biscuitroot species host a variety of insects. These insects are a critical food source for greater sage-grouse chicks (Tilley et al. 2011; Ogle et al. 2012). Adult sage-grouse likely select sites with Gray’s biscuitroot as it is common in brood-rearing habitats (Wik 2002; Lambert 2005). In sagebrush communities in Oregon and Nevada, biscuitroot plants are a common food source for female greater sage-grouse (Pennington et al. 2016).
Insects. Bees, moths, and butterflies utilize Gray’s biscuitroot. In northern Utah, miner bees (Andrena candida) are one of the first bees to appear in the spring. Miner bees visit pussy willows (Salix discolor) first. When pussy willow flowers fade, Gray’s biscuitroot becomes their primary host (Youssef and Bohart 1968). Moths (Greya subalba) oviposit in immature Gray’s biscuitroot schizocarps (Thompson 1987a), and Gray’s biscuitroot is a host plant for Indra swallowtail (Papilio indra) and anise swallowtail (P. zelicaon) (Ogle et al. 2019). Indra swallowtail is a western species that prefers laying eggs on 21 species of Apiaceae, 12 of which are biscuitroots (Dev et al. 2007). In eastern Washington, anise swallowtail females laid an average of 80 to 90% of their eggs on Gray’s biscuitroot or rockloving wavewing (Pteryxia petraea) (Thompson 1988b).
In controlled studies, Gray’s biscuitroot was used to evaluate host preference of Indra and anise swallowtails. Two female anise swallowtails captured from Yakima County, Washington, that were confined with Gray’s biscuitroot laid 15 eggs in 2 days. Female Indra swallowtails captured in Klickitat County, Washington, confined with Gray’s biscuitroot laid 7 eggs in 4 days (James and Nunnallee 2011). Anise swallowtail butterflies from four geographically isolated western populations showed a consistently high preference for Gray’s biscuitroot. Even two of the anise swallowtail populations lacking Gray’s biscuitroot in their natural habitats, oviposited in Gray’s biscuitroot (Thompson 1993). In another study, anise and Oregon swallowtails (P. oregonius) were collected from the field and allowed to oviposit in host plants (tarragon [Artemisia dracunculus] for Oregon swallowtails; Gray’s biscuitroot or rockloving wavewing for anise swallowtail). Larvae were reared on these host plants, and emerging females were presented with a variety of host plants including tarragon, Gray’s biscuitroot, rockloving wavewing, and fennel (Foeniculum spp.). Less than 5% of Oregon swallowtail and about 40% of anise swallowtail eggs were laid on Gray’s biscuitroot. Findings revealed that female swallowtails preferred to oviposit in their host plants (Thompson 1988a).
Ethnobotany
Gray’s biscuitroot was an important food for many Indigenous people. Roots were often cooked, dried and ground into a powder, and mixed with cereals or added to soups. Tender young stems are eaten raw, and greens are used to flavor cooked foods (Marshall 1977; Hunn and French 1981).
Some tribes relied on Gray’s biscuitroot in the spring while others ate it when other foods were scarce (USDA FS 1937; COSEWIC 2008; USU Ext. 2017). Gray’s biscuitroot sprouts were harvested in late winter and early spring, and roots of plants with well developed tops were harvested later. The root harvest rate was an estimated 9 lbs (4 kg)/hour, so a year’s supply of roots for a family of four would take approximately 400 hours (Hunn and French 1981). Gray’s biscuitroot provides a valuable source of vitamin C (15 mg/g per 100 g of edible sprout portion) (Norton et al. 1984).
Gray’s biscuitroot was an important early season, vitamin C-rich food for the Plateau Sahaptin people throughout the Columbia Basin (personal communication cited in COSEWIC 2008). Many Sahaptin-speaking natives residing in the Columbia Plateau relied on biscuitroot species as a primary food source (Hunn and French 1981). The Klikitat people of south-central Washington harvested Gray’s biscuitroot in late winter or early spring (Norton et al. 1999). The Paiute of Oregon harvested and ate young stems raw and stored and ate roots when hungry in the winter (Moerman 2003). The Nez Perce collected and ate biscuitroot stems in March and April before plants flowered (Marshall 1977).
Horticulture
Gray’s biscuitroot was not noted as a landscape plant in the literature. However, its long lifespan, drought tolerance, and attractive early flowers that are important to pollinators (Thompson 1987b; Taylor 1992; Ogle et al. 2019) suggest it could be a valuable addition to low-maintenance landscapes.
Revegetation Use
Gray’s biscuitroot is long-lived, competitive with weeds once established, and provides important early season forage for livestock, wildlife, and pollinators, making it a potentially valuable component of wildland restoration seed mixes (Nye 1980; Thompson 1987b; Cane 2004; Ogle and Brazee 2009; Tilley et al. 2011). Gray’s biscuitroot is recommended for sites receiving 12 to 20 in (305–508 mm) of annual precipitation. Seedlings grow slowly (Ogle et al. 2019), and this trait will require consideration when evaluating restoration goals and developing restoration strategies.
Developing A Seed Supply
For restoration to be successful, the right seed needs to be planted in the right place at the right time. Coordinated planning and cooperation is required among partners to first select appropriate species and seed sources and then properly collect, grow, certify, clean, store, and distribute seed for restoration (PCA 2015).
Developing a seed supply begins with seed collection from native stands. Collection sites are determined by current or projected revegetation requirements and goals. Production of nursery stock requires less seed than large-scale seeding operations, which may require establishment of agricultural seed production fields. Regardless of the size and complexity of any revegetation effort, seed certification is essential for tracking seed origin from collection through use (UCIA 2015).
Seed Sourcing
Because empirical seed zones are not currently available for Gray’s biscuitroot, generalized provisional seed zones developed by Bower et al. (2014), may be used to select and deploy seed sources. These provisional seed zones identify areas of climatic similarity with comparable winter minimum temperature and aridity (annual heat:moisture index). In Figure 7, Omernik Level III Ecoregions (Omernik 1987) overlay the provisional seed zones to identify climatically similar but ecologically different areas. For site-specific disturbance regimes and restoration objectives, seed collection locations within a seed zone and ecoregion may be further limited by elevation, soil type, or other factors.
The Western Wildland Environmental Threat Assessment Center’s (USFS WWETAC 2017) Threat and Resource Mapping (TRM) Seed Zone application provides links to interactive mapping features useful for seed collection and deployment planning. The Climate Smart Restoration Tool (Richardson et al. 2020) can also guide revegetation planning, seed collection, and seed deployment, particularly when addressing climate change considerations.
Occurrence Map
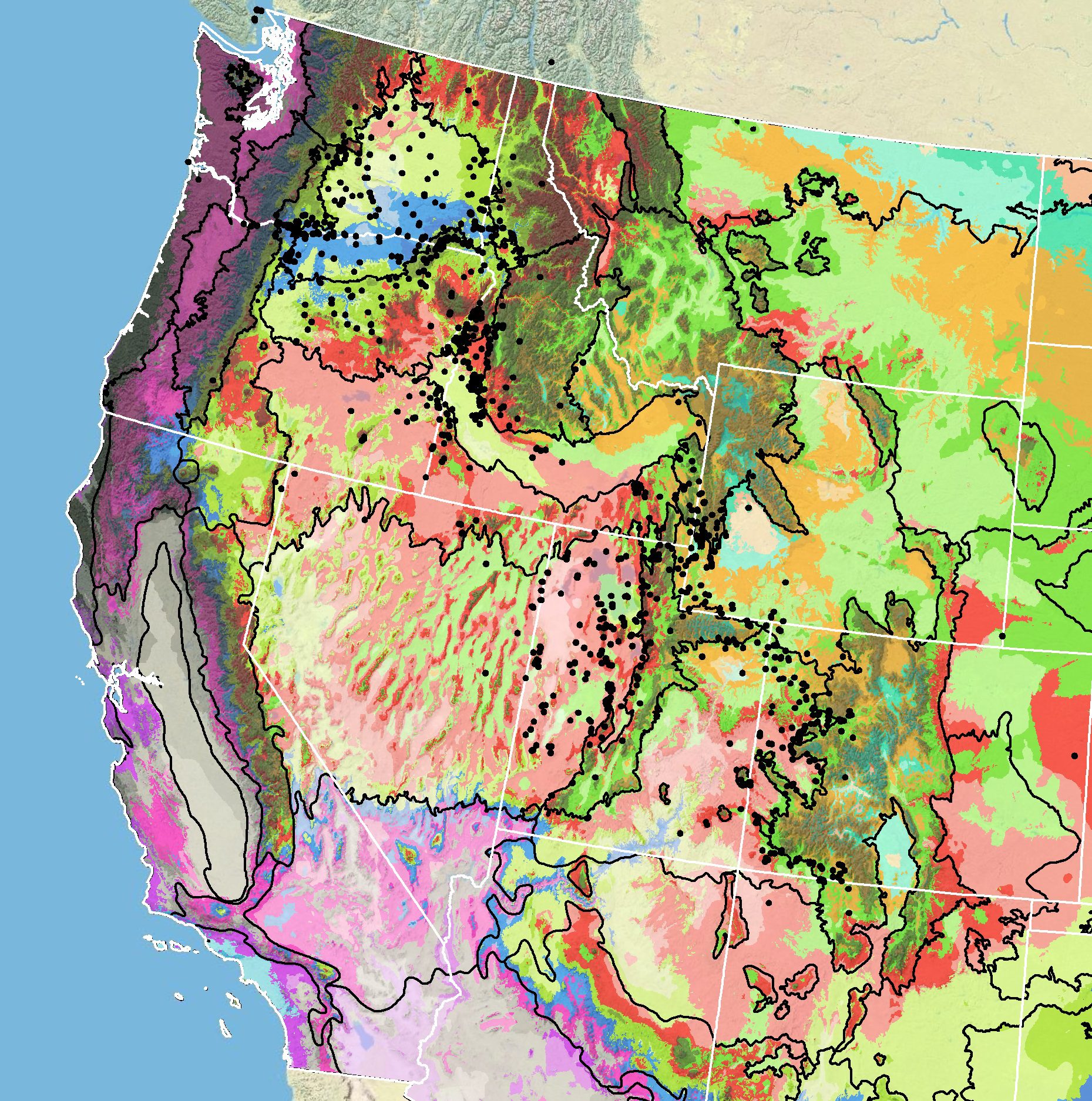
Figure 7. Distribution of Gray’s biscuitroot (black circles) based on geo-referenced herbarium specimens and observational data from 1881-2016 (CPNWH 2017; SEINet 2017; USDI USGS 2017). Generalized provisional seed zones (colored regions) (Bower et al. 2014) are overlain by Omernik Level III Ecoregions (black outlines) (Omernik 1987; USDI EPA 2018). Interactive maps, legends, and a mobile app are available (USFS WWETAC 2017; www.fs.fed.us/wwetac/threat-map/TRMSeedZoneMapper2.php?). Map prepared by M. Fisk, USDI USGS.
Releases
As of 2020, there were no Gray’s biscuitroot germplasm releases.
Wildland Seed Collection
Gray’s biscuitroot seed is collected when the schizocarps are dry, brown with parchment colored wings, and they readily detach from the umbel (Fig. 8). Seed is easily collected by hand stripping fruits from the umbel (Parkinson and DeBolt 2005). State seed laboratories do not distinguish between schizocarps and mericarps when referring to the pure seed unit. In the following sections, the term seed refers to either schizocarps or mericarps.
Figure 8. Gray’s biscuitroot compound umbels with schizocarps approaching maturity. Photo: BLM OR030 SOS.
Wildland Seed Certification
Verification of species and tracking of geographic source is necessary whether wildland seed is collected for immediate project use or as stock seed for cultivated increase. This official Source Identification process can be accomplished by following procedures established by the Association of Official Seed Certifying Agencies (AOSCA) Pre-Variety Germplasm Program (UCIA 2015; Young et al. 2020). Wildland seed collectors should become acquainted with state certification agency procedures, regulations, and deadlines in the states where they collect.
If wildland-collected seed is to be sold for direct use in ecological restoration projects, collectors must apply for Source-Identified certification prior to making collections. Pre-collection applications, site inspections, and species and seed amount verification are handled by the AOSCA member state agency where seed collections will be made (see listings at AOSCA.org).
If wildland seed collected by a grower or private collector is to be used as stock seed for planting cultivated seed fields or for nursery propagation (See Agricultural Seed Field Certification section), detailed information regarding collection site and collecting procedures must be provided when applying for certification. Photos and herbarium specimens may be required. Germplasm accessions acquired within established protocols of recognized public agencies, however, are normally eligible to enter the certification process as stock seed without routine certification agency site inspections. For contract grow-outs, however, this collection site information must be provided to the grower to enable certification.
Collection Timing
Seed is ready for harvest when schizocarps are brown and dry and easily detached from the inflorescence, which typically occurs 5 to 8 weeks after flowering depending on location and weather conditions (Parkinson and DeBolt 2005). Most seed collections made by the Bureau of Land Management’s Seeds of Success (SOS) occurred in June or July (USDI BLM SOS 2017). There were 14 SOS harvests made in Washington, Oregon, Idaho, Utah, and Colorado between 2002 and 2016. Two harvests were made in May, seven in June, and five in July. The earliest collection was made on May 22, 2013 at 3,415-ft (1,041 m) elevation in Malheur County, Oregon. The latest collection was made on July 20, 2010 at 6,631-ft (2,021 m) elevation in Tooele County, Utah. In 2011, there were four collections made. The earliest was on June 7 in Malheur County, Oregon, at an elevation of 2,308 ft (703 m), and the latest was on June 27 in Adams County, Idaho, at an elevation of 2,034 ft (620 m) (USDI BLM SOS 2017), suggesting that environmental conditions besides elevation are important to seed maturation.
Collection Methods
Wildland seed is easily hand collected because seed readily disarticulates from the umbels (Fig. 9) and can be hand stripped from the umbels into a container (Parkinson and DeBolt 2005). Very clean small collections can be made by shaking ripened inflorescences over a bag or tarp (Tilley et al. 2011; Ogle et al. 2012).
Figure 9. Gray’s biscuitroot schizocarps and mericarps from a single umbellet. Photo: BLM ID931 SOS.
Several collection guidelines and methods should be followed to maximize the genetic diversity of wildland collections: 1) collect seed from a minimum of 50 randomly selected plants; 2) collect from widely separated individuals throughout a population without favoring the most robust or avoiding small stature plants; and 3) collect from all microsites including habitat edges (Basey et al. 2015). General collecting recommendations and guidelines are provided in online manuals (e.g., ENSCONET 2009; USDI BLM SOS 2021).It is critical that wildland seed collection does not impact the sustainability of native plant populations. Collectors should take no more than 20% of the viable seed available at the time of harvest (USDI BLM SOS 2021). Additionally, care must be taken to avoid the inadvertent collection of weedy species, particularly those that produce seeds similar in shape and size to those of Gray’s biscuitroot.
Post-Collection Management
Seed should be kept in a dry, shaded place until it can be moved to a controlled short-term storage environment. Short-term storage should be in a dry, cool location inaccessible to rodents or other seed predators. If insects are suspected in any collection, seed should be frozen for 48 hours or treated with an appropriate insecticide. The more plant material in the collection, the more ventilation and drying a seed lot may need (Gold n.d.; Parkinson and DeBolt 2005; Hay and Probert 2011).
Seed Cleaning
Small lots of hand-collected Gray’s biscuitroot seed can be cleaned with minor sieving and air screening (Fig. 10). Tilley et al. (2011) reported purities of nearly 100% using an air screen cleaner to remove stems, unfilled seed, and chaff. Parkinson and DeBolt (2005) carefully rubbed dry seeds through a 0.5-in (1.3 cm) square screen to remove large debris and then through a sieve with 2.36 mm square openings (No. 8 USA STS) to remove fine debris. Careful rubbing is necessary because seeds are brittle and break under too much pressure. Seeds were then spread over a light table and unfilled seeds were discarded, which is an optional method for checking seed fill for nursery production (Parkinson and DeBolt 2005).
At the Bend Seed Extractory, a small (2.6 lb [1.2 kg]), hand-collected, wildland seed lot was carefully rubbed, air-screened, and then processed through an office clipper. Fruits were carefully rubbed by hand to remove seeds, then air-screened to remove non-seed material. Seed was then processed using a Clipper Eclipse, Model 324 (Hoffman Mfg., Corvallis, OR) first with a 24 then a 23 top screen, a 22 round middle screen, a 6 round bottom screen, and low air. The seed lot was again air-screened using an office Clipper (Hoffman Mfg., Corvallis, OR) with a 24 round top screen, 6 round bottom screen, medium speed, and medium air (Barner 2008).
Seed Storage
Gray’s biscuitroot seed is orthodox. Seed viability was 78% for dry seed that was frozen for 52 weeks at -4˚F (-20˚C) at Royal Botanic Gardens Kew (RBG Kew 2020). Seed stored by the Bend Seed Extractory was kept at 33 to 38˚F (0.6-3.3˚C) (Barner 2008). Other Lomatium spp. lose viability after 3 to 4 years of storage (Stevens and Monsen 2004).
Seed Testing. Seed fill can be evaluated by viewing mericarps over a light table (Parkinson and DeBolt 2005) or by x-raying.
Figure 10. Close up of clean, dry Gray’s biscuitroot seeds (mericarps). Scale is mm. Photo: BLM OR931 SOS.
Viability Testing
Because no Association of Official Seed Analysts (AOSA 2010) tetrazolium chloride viability procedure exists for biscuitroot species, viability testing procedures should follow those developed for the Apiaceae family. Seed is first imbibed on moist media for 16 hours at 68 to 77˚F (20–25˚C). While not specifically tested for this species, deep morphophysiological seed dormancy has been reported in many other lomatium species (Pelton 1956; Scholten et al., 2009; Kildisheva et al. 2018). Because of this, Gray’s biscuitroot seed should be clipped and soaked in giberillic acid (GA3) overnight before staining. Seed is then cut laterally or longitudinally and exposed to tetrazolium concentrations of 0.1 or 1% at 68 to 95˚F (20–35˚C) for 4 hours or overnight (AOSA 2010). For seed to be viable, the embryo and endosperm will stain and all essential structure be present and undamaged (Vankus, USFS, personal communication, August 2020).
Germination Testing
There is no AOSA procedure for germination testing of Gray’s biscuitroot. Vankus (2006) found that fresh Gray’s biscuitroot seed requires 28 to 63 days of cold stratification at 37 to 41˚F (3–5˚C) to relieve dormancy. Seeds are then incubated on moist blotter paper at 59 to 77˚F (15–25˚C). First germination counts are made at 7 days and the last at 28 days.
Germination Biology
Gray’s biscuitroot seed requires cold exposure to germinate, duration of cold stratification likely varies by seed lot. Karrfalt and Vankus (2007) found that seed germinates without cold stratification when kept at low temperatures (41–50˚F [5–10˚C]), but at warmer temperatures even with cold overnight temperatures, at least 4 weeks of cold stratification (37˚F [3˚C]) was required. Seeds germinate with or without light. Shaw and DeBolt (2005) reported that seed requires about 8 weeks of cold stratification to germinate and that germination occurred at low temperatures (< 50˚F [10˚C]). Gibberellic acid treatments did not overcome seed dormancy. Cox (2016) found that germination was unaffected by smoke treatments using smoke water concentrations of 1:10, 1:100, or 1:1000.
Parkinson and DeBolt (2005) tested germination of filled seeds following storage at room temperature for 18 months. Seeds were soaked in water for 24 hours, then kept on moist blotter paper in a dark germinator at 39˚F (4˚C) for 60 days. Germination began after 35 days in the cold, and after 60 days germination was 78% (Parkinson and DeBolt 2005).
Wildland Seed Yield And Quality
Post-cleaning seed yield and quality of Gray’s biscuitroot seed lots collected in the Intermountain region are provided in Table 1 (USFS BSE 2017). The results indicate that Gray’s biscuitroot seed can generally be cleaned to high levels of purity but that seed fill and viability is variable. Unfilled mericarps are common for wildland collected seed, and state seed testing laboratories do not sort out unfilled seed before determining viability. Average number of seeds/lb reported elsewhere in the literature (28,747-45,000 seeds/lb [63,375-99,206 seeds/ha]) generally fall within the ranges reported in Table 1 (Lambert 2005; Vankus 2006; Barner 2008; RBG Kew 2020), but 100,000 seeds/lb (220,459 seeds/kg) have also been reported (Parkinson and DeBolt 2005).
Table 1. Seed yield and quality of Gray’s biscuitroot seed lots collected in the Intermountain region, cleaned by the Bend Seed Extractory, and tested by the Oregon State Seed Laboratory or the USFS National Seed Laboratory (USFS BSE 2017).
Seed lot characteristic |
Mean |
Range |
Samples (no.) |
Bulk weight (lbs) |
1.38 |
0.37–2.60 |
9 |
Clean weight (lbs) |
0.58 |
0.10–1.47 |
9 |
Clean-out ratio |
0.46 |
0.24–0.79 |
9 |
Purity (%) |
94 |
85–99 |
9 |
Fill (%)¹ |
76 |
52–95 |
9 |
Viability (%)² |
86 |
73–96 |
8 |
Seeds/lb |
46,461 |
26,000–56,000 |
9 |
Pure live seeds/lb |
38,636 |
21,965–48,272 |
8 |
¹100 seed X-ray test
²Tetrazolium chloride test
Marketing Standards
Acceptable seed purity, viability, and germination specifications vary with revegetation plans. Purity needs are highest for precision seeding equipment used in nurseries, while some rangeland seeding equipment handles less clean seed quite well.
Agricultural Seed Production
Trials for growing Gray’s biscuitroot for seed production were conducted at the Aberdeen, Idaho, Plant Materials Center (PMC) (Tilley et al. 2011; Ogle et al. 2012) and Oregon State University’s Malheur Experiment Station (OSU MES) (Fig. 11; Shock et al. 2016, 2018). Aboveground growth was slow in the first year, and most plants produced seed by their second or third year (Ogle et al. 2012; Shock et al. 2018). At OSU MES, Gray’s biscuitroot produced reasonable seed yields in the third year, and plants were maintained and harvested for 11 years (Shock et al. 2018). Annual seed yield ranged from 300 to 1,200 lb/ac (336–1,345 kg/ha) depending on the year and generally increased with irrigation (see Irrigation section below [Shock et al. 2016, 2018]).
Figure 11. Gray’s biscuitroot growing in irrigation trial plots at Oregon State University, Malheur Experiment Station near Ontario, OR. Fruits are mostly fully developed but have not dried. Photo: USFS.
Agricultural Seed Certification
In order to minimize genetic changes in specific accessions of native species when increased in cultivated fields, it is essential to track the geographic source and prevent inadvertent hybridization or selection pressure. This is accomplished by following third party seed certification protocols for Pre-Variety Germplasm (PVG) as established by the Association of Official Seed Certification Agencies (AOSCA). AOSCA members in the U.S., Canada, and other countries administer PVG requirements and standards that track the source and generation of planting stock. Field and cleaning facility inspections then monitor stand establishment, proper isolation distances, control of prohibited weeds, seed harvesting, cleaning, sampling, testing, and labeling for commercial sales (UCIA 2015; Young et al. 2020).
Seed growers apply for certification of their production fields prior to planting and plant only certified stock seed of an allowed generation (usually less than four). The systematic and sequential tracking through the certification process requires preplanning, knowing state regulations and deadlines, and is most smoothly navigated by working closely with state certification agency personnel. See the Wildland Seed Certification section for more information on stock seed sourcing.
Site Preparation
A firm, weed-free seedbed should be prepared for planting.
Weed Management
A variety of weed control methods were tested and used in combination in seed production plots at the Aberdeen PMC and OSU MES. Weeds can be controlled by using weed barrier fabric, hand-rouging, and pre- and post-emergent herbicides (Shock et al. 2011; Ogle et al. 2012). At the Aberdeen PMC, seed production plots were hand weeded and herbicide treated. Plots were wick treated with glyphosate when Gray’s biscuitroot was actively growing and foliar treated with glyphosate after plants had senesced in early summer (St. John and Ogle 2011; Tilley et al. 2011; Ogle et al. 2012).
Trials at OSU MES evaluated emergence with preemergent herbicide treatments and seed production following post-emergent herbicide treatments. Most preemergent herbicide treatments had minimal effect on emergence, which was low overall (Table 2; Shock et al. 2011). In preemergence herbicide trials, Gray’s biscuitroot was seeded (30 viable seeds/ft [98/m] of row) on December 1, 2009. Seed was placed on the soil surface then covered with a thin layer of sawdust (0.18 oz/ft [0.6 g/m] of row) and row cover until April 7, 2010 (Shock et al. 2011).
Table 2. Emergence of Gray’s biscuitroot in spring 2010 following preemergence herbicide treatments in fall 2009 in experimental plots at Oregon State University’s Malheur Experiment Station, Ontario, OR (Shock et al. 2011).*There were no significant differences between treatments of pendimethalin with and without activated charcoal.
Herbicide, applied Nov. 30, 2009 |
Application rate (lb ai/ac) |
Emergence (%) |
Control |
4.0 |
|
Bensulide |
5.0 |
3.7 |
Pronamide |
1.0 |
28.2 |
Trifluralin |
0.375 |
5.3 |
Pendimethalin |
0.75 |
4.2 |
Benefin |
1.2 |
2.8 |
Dimethenamid |
0.656 |
2.2 |
Linuron |
0.5 |
2.8 |
Seed yield of Gray’s biscuitroot was relatively unaffected by annually repeated post-emergent herbicide treatments (Table 3). Herbicides were applied in the spring for three consecutive years. Researchers caution that seed yields represent small, one-row plots without borders (Shock et al. 2011).
Table 3. Seed yield of Gray’s biscuitroot following the third year of post-emergent herbicide treatments in experimental plots at Oregon State University’s Malheur Experiment Station, Ontario, OR (Shock et al. 2011).
Herbicide |
Application rate (lb ia/ac) |
Mar 20, 2009 |
Seed yields (lbs/ac) |
||
Control |
NA |
32.6 |
Bromoxynil |
0.125 |
20.8 |
Oxyfluorfen |
0.125 |
28.2 |
Clethodim |
0.094 |
38.2 |
Pendimethalin |
1.0 |
28.6 |
Prometryn |
0.8 |
27.8 |
Dimethenamid |
0.656 |
26.6 |
Linuron |
0.5 |
31.2 |
Regular fertilization and herbicide treatments were applied to maintain Gray’s biscuitroot seed production stands seeded for evaluation of irrigation treatments (Table 4). Nitrogen and other nutrients were delivered through the buried drip irrigation system, except for the November 11, 2006 application which was broadcast. All herbicide treatments were broadcast in spray or powder form (Shock et al. 2018).
Table 4. Fertilization and herbicide treatments applied (per ac) to maintain Gray’s biscuitroot seed production stands seeded in fall 2005 at Oregon State University’s Malheur Experiment Station in Ontario, OR. Treatments with more than one date were applied twice at the rate provided (Shock et al. 2018).
Treatment date |
Fertilizer |
Herbicide |
||||||||
Year |
Fertilizer |
Herbicide |
N |
P |
Zn |
Fe |
P |
C |
G |
S |
lbs/ac |
lbs ai/ac |
|||||||||
2006 |
Oct 27, Nov 11 |
Nov 17 |
100 |
50 |
2 |
1 |
||||
2007 |
Nov 9 |
1 |
||||||||
2008 |
Apr 15 |
1 |
||||||||
2009 |
Apr 8 |
Mar 18, Dec 4 |
50 |
10 |
2 |
0.5 |
||||
2010 |
Nov 17 |
1 |
||||||||
2011 |
May 3 |
Nov 9 |
50 |
1 |
||||||
2012 |
Apr 13 |
Nov 7 |
50 |
10 |
0.3 |
1 |
||||
2013 |
Mar 29 |
Apr 3 |
20 |
25 |
0.3 |
2 |
||||
2014 |
Apr 2 |
Feb 26 |
20 |
25 |
0.3 |
1 |
2 |
|||
2015 |
Apr 15 |
Mar 13, Nov 6 |
20 |
25 |
0.3 |
2 |
1.5 |
|||
2016 |
Mar 31 |
Oct 27 |
20 |
25 |
0.3 |
1 |
||||
2017 |
Apr 4 |
Mar 28 |
0.3 |
1 |
0.75 |
*P=pendimethalin, C=clethodim, G=glyphosate, S= Sethoxydim
Seeding
Gray’s biscuitroot seed requires cold stratification to germinate. At OSU MES, seed that was cold stratified (about 5 wks at 34˚F [1˚C]) and seeded on March 3, resulted in an uneven stand, but Gray’s biscuitroot seed planted on October 26 and allowed to stratify naturally resulted in excellent stands the following spring (Shock et al. 2018).
Based on research at the Aberdeen PMC, seed production fields should be seeded at 25 to 30 PLS/linear ft (82–98/m), and rows planted on 30-in (75 cm) centers to allow for between row cultivation and weed control. When using weed barrier fabric, seeds were planted in 3-in (8 cm) holes spaced 12 to 18 in (30–45 cm) apart (Tilley et al. 2011; Ogle et al. 2012). Gray’s biscuitroot that was direct seeded on November 2005 at the Aberdeen PMC had 70% survival on June 20, 2007 (St. John and Ogle 2011).
At OSU MES, Gray’s biscuitroot was seeded on October 26, 2005 in Nyssa silt loam soil using a custom, small-plot, grain drill with disk openers. Seed was planted 0.5 in (1.3 cm) deep at 20 to 30 PLS/ft (66–98/m) of row. In spring 2006, stands were considered excellent (Shock et al. 2016).
Establishment And Growth
In its first year, Gray’s biscuitroot has limited aboveground growth and allocates an abundance of resources to its taproot. Gray’s biscuitroot seedlings grow faster than fernleaf biscuitroot (Lomatium dissectum) and nineleaf biscuitroot (L. triternatum) (Tilley et al. 2011; Ogle et al. 2012). At OSU MES, first flowering of seedings made in fall 2005 occurred in 2007. Over an 11-year period, initiation of flowering occurred from early March through mid-May. Seed matured in June (Table 5).
Table 5. Timing of flowering and seed harvest dates for Gray’s biscuitroot seed production fields over a period of 9 years at Oregon State University’s Malheur Experiment Station, Ontario, OR (Shock et al. 2016, 2018). Seed yields are provided in Table 7.
Year |
Flower start |
Flower end |
Harvest |
———————- Date———————- |
|||
2007 |
5 Apr |
10 May |
30 May, 29 Jun |
2008 |
25 Mar |
15 May |
30 May, 19 Jun |
2009 |
10 Mar |
7 May |
16 Jun |
2010 |
15 Mar |
15 May |
22 Jun |
2011 |
1 Apr |
13 May |
22 Jun |
2012 |
15 Mar |
16 May |
14 Jun |
2013 |
15 Mar |
30 Apr |
10 Jun |
2014 |
28 Mar |
2 May |
10 Jun |
2015 |
1 Mar |
28 Apr |
1 Jun |
2016 |
7 Mar |
29 Apr |
1 Jun |
2017 |
15 Mar |
12 May |
8 Jun |
Irrigation
Trials at OSU MES found that plant size and seed yields of Gray’s biscuitroot were significantly better with irrigation (Shock et al. 2016, 2018). Irrigation trials began in March 2006 for plots seeded in late October 2005. Irrigation treatments of 0, 4, or 8 in (101 or 203 mm) were delivered four times at 2-week intervals beginning at the time of bud formation and flowering. Irrigation was delivered through drip tape buried 12 in (30 cm) deep, so as not to affect flowering and to limit germination of weed seed (Shock et al. 2016). In most years, Gray’s biscuitroot seed yields increased significantly with irrigation (P < 0.05). Seed yields averaged over 11 years were 438 lbs/ac (491 kg/ha) with 0 in, 719 lbs/ac (806 kg/ha) with 4 in (100 mm), and 622 lbs/ac (697 kg/ha) with 8 in (200 mm) of supplemental irrigation. Seed yield showed no significant response to irrigation in 2010 and 2011, when precipitation (spring, winter, and fall) was above average (Table 6; Shock et al. 2016, 2018).
Table 6. Precipitation (in) and growing degree hours (°F) at Oregon State University’s Malheur Experiment Station in Ontario, OR (Shock et al. 2016, 2018).
Year |
Spring |
Winter + Spring |
Fall + Winter + Spring |
Growing degree hrs (50-86 °F) Jan-June |
2006 |
3.4 |
10.1 |
14.5 |
1273 |
2007 |
1.9 |
3.8 |
6.2 |
1406 |
2008 |
1.4 |
3.2 |
6.7 |
1087 |
2009 |
4.1 |
6.7 |
8.9 |
1207 |
2010 |
4.3 |
8.4 |
11.7 |
971 |
2011 |
4.8 |
9.3 |
14.5 |
856 |
2012 |
2.6 |
6.1 |
8.4 |
1228 |
2013 |
0.9 |
2.4 |
5.3 |
1319 |
2014 |
1.7 |
5.1 |
8.1 |
1333 |
2015 |
3.2 |
5.9 |
10.4 |
1610 |
2016 |
2.2 |
5.0 |
10.1 |
1458 |
2017 |
4.0 |
9.7 |
12.7 |
1196 |
12-yr ave |
2.9 |
6.3 |
9.8 |
1207 (25-yr ave) |
Pollinator Management
Gray’s biscuitroot is pollinated by early emerging solitary bees and flies (Nye 1980; Cane 2004). In Green Canyon, Cache County, Utah, sweat bees visited Gray’s biscuitroot for nectar and pollen in April and May (Nye 1980). Any cultural practices to encourage early emerging pollinators may benefit seed production fields.
Table 7. Seed yields (lbs/ac) for Gray’s biscuitroot growing with and without supplemental irrigation at Oregon State University’s Malheur Experiment Station near Ontario, OR. Gray’s biscuitroot was seeded in fall 2005 (Shock et al. 2018).
Year* | Supplemental irrigation | ||
0 in | 4 in | 8 in | |
Seed yield (lbs/ac) | |||
2007 | 36.1a | 88.3ab | 131.9b |
2008 | 393.3a | 1287.0b | 1444.9c |
2009 | 359.9a | 579.8b | 686.5b |
2010 | 1035.7a | 1143.5a | 704.8a |
2011 | 570.3a | 572.7a | 347.6a |
2012 | 231.9a | 404.4b | 377.3b |
2013 | 596.7a | 933.4a | 1036.3a |
2014 | 533.1a | 1418.1b | 1241.3b |
2015 | 186.4a | 576.7b | 297.6a |
2016 | 483.7ab | 644.2b | 322.9a |
2017 | 333.5a | 259.5a | 246.3a |
Mean | 438.4a | 718.9b | 621.6ab |
Values within the same row followed by different letters are significantly different (P < 0.05).
Pest Management
Insects, mammals, and fungi may all potentially have deleterious impacts on Gray’s biscuitroot growth and reproduction. The negative impacts of insects and mammals on plant growth and reproduction are well known (see Wildlife and Livestock Use section). However, less is known about the fungi. The following fungi were collected from wild populations of Gray’s biscuitroot: Ascochyta lomatii, Pleospora scrophulariae, Phyllachora spp., Puccinia jonesii, Ramularia heraclei (Cooke 1952; Farr and Rossman 2017), but infections and impacts in seed production fields were not reported.
At OSU MES, mowing plants after they went dormant in early fall of each year reduced vole (Cricetidae) damage (Shock et al. 2018).
Seed Harvesting
Gray’s biscuitroot seed can be harvested in production fields with a vacuum type harvester or flail vac (Tilley et al. 2011). The Aberdeen PMC developed the Jet Harvester which has been used to harvest Gray’s biscuitroot seed. This piece of equipment is more efficient than hand harvesting and requires little post-harvest cleaning (Tilley and Bair 2011).
Seed Yields And Stand Life
At OSU MES, Gray’s biscuitroot seed was harvested over an 11-year period (Shock et al. 2018). Shock et al. (2018) found on average seed production (Fig. 12) was maximized with 14.3 in (363 mm) of water, which included irrigation and fall, winter, and spring precipitation (Table 8). Seed yields were not responsive to irrigation in 2010, 2011, and 2017, years that were cooler and wetter than normal (Tables 6, 7, and 8; Shock et al. 2016, 2018).
Figure 12. Gray’s biscuitroot producing seed at Oregon State University, Malheur Experiment Station near Ontario, OR. Photo taken on June 19, 2008, by USFS.
Table 8. Regression analysis for fernleaf biscuitroot seed yield (y, lbs/ac) in response to irrigation plus spring precipitation (x, mm/season) using equation y=a + b∙x + c∙x² in 2007-2017 and the 11-year average. Study conducted at Oregon State University’s Malheur Experiment Station in Ontario, OR. Only those years where irrigation resulted in significantly greater seed yields are shown below (Shock et al. 2016, 2018).*Not significant.
Year |
Highest yield (lbs/ac) |
Irrigation + fall, winter, and spring precipitation for maximum seed yield (in) |
Spring, winter, fall precipitation (in) |
2007 |
59 |
14.2 |
6.2 |
2008 |
1475 |
13.5 |
6.6 |
2009 |
344 |
16.8 |
8.8 |
2012 |
418 |
13.8 |
8.4 |
2013 |
1075 |
13.3 |
5.3 |
2014 |
1477 |
13.4 |
8.1 |
2015 |
579 |
14.8 |
10.4 |
2016 |
651 |
13.4 |
9.1 |
Ave |
730 |
14.3 |
9.8 |
Nursery Practice
Gray’s biscuitroot can be successfully greenhouse-grown from seed. Ellison and Thompson (1987) seeded Gray’s biscuitroot in late December in trays (16 × 24 × 2 in [41 × 60 × 6 cm]) filled with 0.6 in (1.5 cm) of moist sand. Trays were kept at 41˚F (5˚C) with constant light and watered every 1 to 2 weeks during the first 2 months and every 17 days after that. Seedlings were then transplanted into larger containers filled with a 55% peat and 45% pumice mix with pH near 7. Seedlings were fertilized with NPK 11-12-14 and micronutrients, watered every 2 days, and provided 13 to 14.5 hours of light each day (Ellison and Thompson 1987).
Seed collected in mid- to late-June from sites in southeastern Washington was stored until mid-November after which time, they were placed in trays containing approximately 0.4 in (1 cm) of coarse sand. Seeds were covered with 0.4 in (1 cm) of sand, watered until moist, covered with plastic, and placed in a refrigerator at 39 to 41˚F (4–5˚C). Seeds germinated in January and February. Germinants were placed in 4-in (10 cm) peat pots filled with a 1:1:1, peat, sand, and Palouse loam mix and treated with calcium carbonate to neutralize pH. Seedlings were kept in an unheated greenhouse and fertilized each week with NPK 14-14-14, slow release fertilizer until transplanted in an experimental garden in early to mid-April (Thompson 1987b).
Parkinson and DeBolt (2005) stratified Gray’s biscuitroot seed (39˚F [4˚C]) for 60 days and placed germinated seed in cone-tainers filled with a 50-50 peat-vermiculite mixture. Cone-tainers were kept in the greenhouse at a constant 81˚F (27˚C), watered when soil saturation fell below 80%, and minimally fertilized. First true leaves developed after 14 days. Seedlings remained in the greenhouse environment for 84 days, when survival was about 77% (Parkinson and DeBolt 2005).
Wildland Seeding And Planting
Seeding Gray’s biscuitroot is recommended for wildland sites receiving 12 to 20 in (305–508 mm) of annual precipitation (Ogle et al. 2019). Seed can be broadcast or drill seeded to a maximum depth of 0.5 in (1.3 cm) in the fall at a pure seeding rate of 20 to 24 PLS lbs/ac (22-27 kg/ha) (Ogle et al. 2012, 2017; Tilley et al. 2011). Pure seeding rates should be adjusted to represent the desired percentage of the seed mix (Tilley et al. 2011). Thorough evaluations of seeding and planting efforts for the restoration of Gray’s biscuitroot in degraded areas are needed. Impacts of herbivores, granivores, and competition from invasive species will likely pose a challenge to restoration using Gray’s biscuitroot.
Ecological studies suggest that Gray’s biscuitroot is an opportunistic species that can colonize old fields and openings created by herbicide treatments. In the Columbia River Basin of eastern Washington, Gray’s biscuitroot naturally colonized 57-year-old abandoned agriculture fields in the big sagebrush/bluebunch wheatgrass habitat type. The frequency of Gray’s biscuitroot was 40 to 55% and its density was up to 20 plants/500 m² at warm, dry parts of the old field (7.7 in [195 mm] annual ppt., 54.5˚F [12.5˚C] maximum Jan. temp., 4.1˚F [-15.5˚C] minimum Jan. temp.). At wetter and cooler parts of the old field, Gray’s biscuitroot was a common colonizer (8.9 in [225 mm] annual ppt., 52.7˚F [11.5˚C] maximum Jan. temp., 1.4˚F (-17˚C) minimum Jan. temp.) (Simmons and Rickard 2002). On private property within the Cache National Forest in Utah, Gray’s biscuitroot replaced timber mildvetch (Astragalus miser) as the dominant forb on herbicide-treated plots (2,4,5-TES, 4-MCPB, and 2,4-DB) (Cronin and Coburn 1964).
Acknowledgements
Funding for Western Forbs: Biology, Ecology, and Use in Restoration was provided by the USDI BLM Great Basin Native Plant Materials Ecoregional Program through the Great Basin Fire Science Exchange. Great thanks to the chapter reviewers: Lauren Svejcar, PhD Student, Murdoch University and Olga Kildisheva, Project Manager, The Nature Conservancy.
This research was supported in part by the USDA Forest Service, Rocky Mountain Research Station. The findings and conclusions in this publication are those of the authors and should not be construed to represent any official USDA or U.S. Government determination or policy.
Literature Cited
Andersen, B.A.; Holmgren, A.H. 1996. Mountain plants of northeastern Utah. Logan, UT: Utah State University, Extension Services. 148 p.
Association of Official Seed Analysts [AOSA]. 2010. AOSA/SCST Tetrazolium testing handbook. Contribution No. 29. Lincoln, NE: Association of Official Seed Analysts.
Barner, J. 2008. Propagation protocol for production of propagules (seeds) Lomatium grayi (J.M. Coult. & Rose) J.M. Coult. & Rose. Native Plant Network. U.S. Department of Agriculture, Forest Service, National Center for Reforestation, Nurseries, and Genetic Resources. http://npn.rngr.net/propagation/protocols [Accessed 2020 March 5].
Basey, A.C.; Fant, J.B.; Kramer, A.T. 2015. Producing native plant materials for restoration: 10 rules to collect and maintain genetic diversity. Native Plants Journal. 16(1): 37-53.
Bolen, E.G. 1964. Plant ecology of spring-fed salt marshes in western Utah. Ecological Monographs. 34(2): 143-166.
Bower, A.D.; St. Clair, J.B.; Erickson, V. 2014. Generalized provisional seed zones for native plants. Ecological Applications. 24(5): 913-919.
Cane, J.H. 2004. Pollinator and seed predator studies. In: Shaw, N.L.; Pellant, M., eds. Great Basin Native Plant Selection and Increase Project: 2003 Progress Report. Boise, ID: U.S. Department of Agriculture, Forest Service, Rocky Mountain Research Station: 24-26.
Consortium of Pacific Northwest Herbaria [CPNWH]. 2017. Seattle, WA: University of Washington Herbarium, Burke Museum of Natural History and Culture. http://www.pnwherbaria.org/index.php
Cooke, W.B. 1952. Western fungi: II. Mycologia. 44(2): 245-261.
COSEWIC. 2008. Gray’s desert-parsley (Lomatium grayi): COSEWIC assessment and status report 2008. Ottawa, Ontario: Committee on the Status of Endangered Wildlife in Canada. https://www.canada.ca/en/environment-climate-change/services/species-risk-public-registry/cosewic-assessments-status-reports/gray-desert-parsley-2008.html [Accessed 2020 June 19].
Coulter, J.M.; Rose, J.N. 1900. Monograph of the North American Umbelliferae. Systematic and Geographic Botany and Aboriginal Uses of Plants. 7(1): 9-256.
Coulter, J.M.; Rose, J.N. 1909. Supplement to the monograph of the North American Umbelliferae. Systematic Investigations and Bibliography. 12(10): 441-451.
Cox, R. 2016. Smoke-induced germination of Great Basin native forbs. In: Kilkenny, F; Edwards, F.; Malcomb, A., eds. Great Basin Native Plant Project: 2015 Progress Report. Boise, ID: U.S. Department of Agriculture, Forest Service, Rocky Mountain Research Station: 78-82.
Craighead, J.J.; Craighead, F.C. Jr.; Davis, R.J. 1963. A field guide to Rocky Mountain wildflowers from northern Arizona and New Mexico to British Columbia. Boston, MA: Houghton Mifflin Company. 277 p.
Crawford, R.C. 2003. Riparian vegetation classification of the Columbia Basin, Washington. Olympia, WA: Washington State Department of Natural Resources, Washington Natural Heritage Program. 99 p.
Cronin, E.H.; Coburn, M.C. 1964. Chemical control of timber milkvetch and effects on associated vegetation. Weeds. 12(3): 177-179.
Crowe, E.A.; Busacca, A.J.; Reganold, J.P.; Zamora, B.A. 1994. Vegetation zones and soil characteristics in vernal pools in the channeled scabland of eastern Washington. Great Basin Naturalist. 54(3): 234-247.
Dev, V.; Ly, B.; Miranda, A.E.; Whaley, W. 2007. Lomatium grayi and Indra swallowtail butterfly composition of the essential oils of three varieties of Lomatium grayi (J.M. Coult et Rose) J.M. Coult et Rose. Journal of Essential Oil Research. 19: 244-248.
Dyni, E.J.; Yensen, E. 1996. Dietary similarity of sympatric Idaho and Columbian ground squirrels (Spermophilus brunneus and S. columbianus). Northwest Science. 70(2): 99-108.
Ellison, R.L.; Thompson, J.N. 1987. Variation in seed and seedling size: The effects of seed herbivores on Lomatium grayi (Umbelliferae). Oikos. 49(3): 269-280.
European Native Seed Conservation Network [ENSCONET]. 2009. ENSCONET seed collecting manual for wild species. Edition 1: 32 p.
Farr, D.F.; Rossman, A.Y. 2020. Fungal databases, U.S. National Fungus Collections. U.S. Department of Agriculture, Agricultural Research Service. https://nt.ars-grin.gov/fungaldatabases/
Gold, K. n.d. Post-harvest handling of seed collections. Technical Information Sheet 04. UK: Royal Botanic Gardens Kew and Millennium Seed Bank Partnership. 4 p.
Hall, F. 2007. Variation in shrub and herb cover and production on ungrazed pine and sagebrush sites in eastern Oregon: A 27-year photomonitoring study. Gen. Tech. Rep. PNW-GTR-704. Portland, OR: U.S. Department of Agriculture, Forest Service, Pacific Northwest Research Station. 44 p.
Hay F.R.; Probert, R.J. 2011. Chapter 20: Collecting and handling seeds in the field. In: Guarino, L.; Ramanatha, V.; Goldberg, E. Collecting plant genetic diversity: Technical Guidelines-2011 update. Rome, Italy: Bioversity International. 33 p.
Hermann, F. 1966. Notes on western range forbs: Cruciferae through Compositae. Agric. Handb. 293. Washington, DC: U.S. Department of Agriculture, Forest Service. 365 p.
Hitchcock, C.L.; Cronquist, A. 2018. Flora of the Pacific Northwest: An illustrated manual. Second Ed. Giblin, D.E.; Legler, B.S.; Zika, P.F.; Olmstead, R.G., eds. Seattle, WA: University of Washington Press. 882 p.
Hunn, E.S.; French, D.H. 1981. Lomatium: A key resource for Columbia Plateau native subsistence. Northwest Science. 55(2): 87-94.
Huntly, N.; Bangert, R.; Hanser, S.E. 2011. Native and exotic plants of fragments of sagebrush steppe produced by geomorphic processes versus land use. Plant Ecology. 212(9): 1549-1561.
James, D.G.; Nunnallee, D. 2011. Life histories of Cascadia butterflies. Corvallis, OR: Oregon State University Press. 447 p.
Kachergis, E.; Fernandez-Gimenez, M.E.; Rocca, M.E. 2012. Differences in plant species composition as evidence of alternate states in the sagebrush steppe. Rangeland Ecology and Management. 65(5): 486-497.
Karrfalt, R.; Vankus, V. 2007. Development of germination protocols, seed weight, purity, and seed conditioning/cleaning protocols for Great Basin grasses and forbs. In: Shaw, N.L.; Pellant, M., eds. Great Basin Native Plant Selection and Increase Project: 2006 Progress Report. Boise, ID: U.S. Department of Agriculture, Forest Service, Rocky Mountain Research Station: 80-82.
Kartesz, J.T.; The Biota of North America Program (BONAP). 2015. North American Plant Atlas. http://bonap.net/napa
Kildisheva, O.A.; Erickson, T.E.; Madsen, M.D.; Dixon, K.W.; Merritt, D.J. 2019. Seed germination and dormancy traits of forbs and shrubs important for restoration of North American dryland ecosystems. Plant Biology. 21: 458-469.
Lambert, S. 2005. Guidebook to the seeds of native and non-native grasses, forbs and shrubs of the Great Basin. Boise, ID: U.S. Department of the Interior, Bureau of Land Management, Idaho State Office. 136 p.
Love, S.L.; Akins, C.J. 2019. Fourth summary of the native seed germination studies of Norman C Deno: Species with names beginning with letters L through O. Native Plants. 20(3): 279-305.
Marshall, A.G. 1977. Nez Perce social groups: An ecological interpretation. Pullman, WA: Washington State University. Thesis. 194 p.
Mathias, M.E. 1938. A revision of the genus Lomatium. Annals of the Missouri Botanical Garden. 25(1): 225-297.
Moerman, D. 2003. Native American ethnobotany: A database of foods, drugs, dyes, and fibers of Native American peoples, derived from plants. Dearborn, MI: University of Michigan. http://naeb.brit.org
Norton, H.H.; Boyd, R.; Hunn, E. 1999. The Klikitat Trail of south-central Washington: A reconstruction of seasonally used resource sites. In: Boyd, R., ed. Indians, fire, and the land in the Pacific Northwest. Corvallis, OR: Oregon State University: 65-93.
Norton, H.H.; Hunn, E.S.; Martinsen, C.S.; Keely, P.B. 1984. Vegetable food products of the foraging economies of the Pacific Northwest. Ecology of Food and Nutrition. 14(3): 219-228.
Nye, W.P. 1980. Notes on the biology of Halictus (Halictus) farinosus Smith (Hymenoptera: Halictidae). ARR-W-11. Oakland, CA: U.S. Department of Agriculture, Agricultural Research (Western Region), Science and Education Administration. 29 p.
Ogle, D.; Brazee, B. 2009. Estimating initial stocking rates. TN Range No. 3. Boise, ID: U.S. Department of Agriculture, Natural Resources Conservation Service. 39 p.
Ogle, D.; St. John, L.; Stannard, M.; Holzworth, L. 2012. Conservation plant species for the Intermountain West. Plant Materials Technical Note 24. Boise, ID: U.S. Department of Agriculture, Natural Resources Conservation Service. 57 p.
Ogle, D.; Tilley, D.; Cane, J.; St. John, L.; Fullen, K.; Stannard, M.; Pavek, P. 2019. Plants for pollinators in the Intermountain West. Plant Materials Tech. Note 2A. Boise, ID: U.S. Department of Agriculture, Natural Resources Conservation Service. 40 p.
Omernik, J.M. 1987. Ecoregions of the conterminous United States. Map (scale 1:7,500,000). Annals of the Association of American Geographers. 77(1): 118-125.
Parkinson, H.; DeBolt, A. 2005. Propagation protocol for production of container (plugs) Lomatium grayi Coult. & Rose plants. Native Plant Network. U.S. Department of Agriculture, Forest Service, National Center for Reforestation, Nurseries, and Genetic Resources. http://npn.rngr.net/propagation/protocols [Accessed 2020 March 5].
Parkinson, H.A. 2008. Impacts of native grasses and cheatgrass on Great Basin forb development. Bozeman, MT: Montana State University. Thesis. 73 p.
Pavek, P.; Erhardt, B.; Heekin, T.; Old, R. 2012. Forb seedling identification guide for the Inland Northwest: Native, introduced, invasive, and noxious species. Pullman, WA: U.S. Department of Agriculture, Natural Resources Conservation Service, Pullman Plant Materials Center. 144 p.
Pelton, J. 1956. A study of seed dormancy in eighteen species of high altitude Colorado plants. Butler University Botanical Study. 13(1): 74-84.
Pennington, V.E.; Schlaepfer, D.R.; Beck, J.L.; Bradford, J.B.; Palmquist, K.A.; Lauenroth, W.K. 2016. Sagebrush, greater sage-grouse, and the occurrence and importance of forbs. Western North American Naturalist. 76(3): 298-312.
Plant Conservation Alliance [PCA]. 2015. National seed strategy for rehabilitation and restoration 2015-2020. Washington, DC: U.S. Department of the Interior, Bureau of Land Management. 52 p.
Poston, T.M. 1995. Concentrations of radionuclides in terrestrial vegetation on the Hanford Site of potential interest to Native Americans. PNL-10397. Springfield, VA: Pacific Northwest Laboratory, Battelle Memorial Institute. 25 p.
Rice, A.; Glick, L.; Abadi, S.; Einhorn, M.; Kopelman, N.M.; Salman-Minkov, A.; Mayzel, J.; Chay, O.; Mayrose, I. 2015. The Chromosome Counts Database (CCDB) – A community resource of plant chromosome numbers. New Phytologist. 206(1): 19-26.
Richardson, B.; Kilkenny, F.; St. Clair, B.; Stevenson-Molnar, N. 2020. Climate Smart Restoration Tool. https://climaterestorationtool.org/csrt/
Royal Botanic Gardens Kew [RBG Kew]. 2020. Seed Information Database (SID). Version 7.1. http://data.kew.org/sid/ [Accessed 2020 February 16].
Scholten, M.; Donahue, J.; Shaw, N.L.; Serpe, M.D. 2009. Environmental regulation of dormancy loss in seeds of Lomatium dissectum (Apiaceae). Annals of Botany. 103: 1091-1101.
SEINet – Regional Networks of North American Herbaria Steering Committee [SEINet]. 2017. SEINet Regional Networks of North American Herbaria. https://symbiota.org/seinet
Shaw, N.; DeBolt, A. 2005. Development of germination protocols, seed weight, purity, and seed conditioning/cleaning protocols for Great Basin grasses and forbs. In: Shaw, N.L.; Pellant, M., eds. Great Basin Native Plant Selection and Increase Project: 2004 Progress Report. Boise, ID: U.S. Department of Agriculture, Forest Service, Rocky Mountain Research Station: 9-13.
Shock, C.C.; Feibert, E.B.; Saunders, L.D.; Parris, C.; Shaw, N.; Johnson, D.; Bushman, S. 2012. Seed production of Great Basin native forbs subsurface drip irrigation 2006-2011 new plantings, seeding practices, legume seed scarification. In: Shaw, N.; Pellant, M., eds. Great Basin Native Plant Project: 2011 Progress Report. Boise, ID: U.S. Department of Agriculture, Rocky Mountain Research Station: 93-132.
Shock, C.C.; Feibert, E.B.G.; Rivera, A.; Saunders, L.D. 2018. Irrigation requirements for Lomatium seed production in a semi-arid environment. In: Kilkenny, F; Edwards, F.; Irwin, J.; Barga, S., eds. Great Basin Native Plant Project: 2017 Progress Report. Boise, ID: U.S. Department of Agriculture, Forest Service, Rocky Mountain Research Station: 97-110.
Shock, C.C.; Feibert, E.B.G.; Rivera, A.; Saunders, L.D.; Shaw, N.; Kilkenny, F.F. 2016. Irrigation requirements for seed production of five Lomatium species in a semiarid environment. HortScience. 51(10): 1270-1277.
Simmons, S.A.; Rickard, W.H. 2002. Plant succession at the edges of two abandoned cultivated fields on the Arid Lands Ecology Reserve. Northwest Science. 76(1): 85-89.
Skinner, D.M.; Warnick, P.; French, B.; Fauci, M. 2005. More Palouse forbs for landscaping. Pullman, WA: U.S. Department of Agriculture, Natural Resources Conservation Service. 7 p.
St. John, L.; Ogle, D. 2011. Cooperative work between the Great Basin Native Plant Selection and Increase Project and the Aberdeen Plant Materials Center. In: Shaw, N.; Pellant, M., eds. Great Basin Native Plant Selection and Increase Project: 2010 Progress Report. Boise, ID: U.S. Department of Agriculture, Forest Service, Rocky Mountain Research Station: 69-76.
Stevens, R.; Monsen, S.B. 2004. Forbs for seeding range and wildlife habitats. In: Monsen, S.B.; Stevens, R.; Shaw, N.L., comps. Restoring western ranges and wildlands, vol. 2. Gen. Tech. Rep. RMRS-GTR-136-vol-2. Fort Collins, CO: U.S. Department of Agriculture, Forest Service, Rocky Mountain Research Station: 425-466.
Sun, F.J.; Downie, S.R. 2010. Phylogenetic analyses of morphological and molecular data reveal major clades within the perennial, endemic western North American Apiaceae subfamily Apioideae. The Journal of the Torrey Botanical Society. 137(2/3): 133-156.
Taylor, R.J. 1992. Sagebrush country: A wildflower sanctuary. Missoula, MT: Mountain Press Publishing Company. 211 p.
Thompson, J.N. 1983. Selection of plant parts by Depressaria multifidae (Lep., Oecophoridae) on its seasonally-restricted hostplant, Lomatium grayi (Umbelliferae). Ecological Entomology. 8: 203-211.
Thompson, J.N. 1984. Variation among individual seed masses in Lomatium grayi (Umbelliferae) under controlled conditions: Magnitude and partitioning of the variance. Ecology. 65(2): 626-631.
Thompson, J.N. 1987a. Variance in number of eggs per patch: Oviposition behaviour and population dispersion in a seed parasitic moth. Ecological Entomology. 12(3): 311-320.
Thompson, J.N. 1987b. The ontogeny of flowering and sex expression in divergent populations of Lomatium grayi. Oecologia. 72(4): 605-611.
Thompson, J.N. 1988a. Variation in preference and specificity in monophagous and oligophagous swallowtail butterflies. Evolution. 42(1): 118-128.
Thompson, J.N. 1988b. Evolutionary genetics of oviposition preference in swallowtail butterflies. Evolution. 42(6): 1223-1234.
Thompson, J.N. 1993. Preference hierarchies and the origin of geographic specialization in host use in swallowtail butterflies. Evolution. 47(5): 1585-1594.
Thompson, J.N.; Moody, M.E. 1985. Assessing probability of interaction in size-structured populations: Depressaria attack on Lomatium. Ecology. 66(5): 1597-1607.
Tilley, D.; Bair, C. 2011. The Jet Harvester: A shop tool for harvesting forb and shrub seeds. Native Plants Journal. 12(2): 123-127.
Tilley, D.; St. John, L.; Ogle, D.; Shaw, N. 2011. Plant guide for Gray’s biscuitroot (Lomatium grayi) [J.M. Coult. & Rose] J.M. Coult. & Rose). Aberdeen, ID: U.S. Department of Agriculture, Natural Resources Conservation Service. 4 p.
Turesson, G. 1914. Slope exposure as a factor in the distribution of Pseudotsuga taxifolia in arid parts of Washington. Bulletin of the Torrey Botanical Club. 41(6): 337-345.
USDA Forest Service [USDA FS] 1937. Range plant handbook. Washington, DC: U.S. Department of Agriculture, Forest Service. 816 p.
USDA Forest Service, Bend Seed Extractory [USDA FS BSE]. 2017. Nursery Management Information System Version 4.1.11. Local Source Report 34-Source Received. Bend, OR: U.S. Department of Agriculture, Forest Service, Bend Seed Extractory.
USDA Forest Service, Western Wildland Environmental Threat Assessment Center [USFS WWETAC]. 2017. TRM Seed Zone Applications. Prineville, OR: U.S. Department of Agriculture, Forest Service, Western Wildland Environmental Threat Assessment Center. https://www.fs.fed.us/wwetac/threat-map/TRMSeedZoneMapper.php
USDA Natural Resources Conservation Service [USDA NRCS]. 2019. The PLANTS Database. Greensboro, NC: U.S. Department of Agriculture, Natural Resources Conservation Service, National Plant Data Team. https://plants.usda.gov/java
USDI Bureau of Land Management, Seeds of Success [USDI BLM SOS]. 2016. Bureau of Land Management technical protocol for the collection, study, and conservation of seeds from native plant species for Seeds of Success. Washington, DC: U.S. Department of the Interior, Bureau of Land Management, Plant Conservation and Restoration Program. 37 p.
USDI Bureau of Land Management, Seeds of Success [USDI BLM SOS]. 2017. Seeds of Success collection data. Washington, DC: U.S. Department of the Interior, Bureau of Land Management, Plant Conservation and Restoration Program.
USDI Environmental Protection Agency [USDI EPA]. 2017. Ecoregions. Washington, DC: U.S. Department of the Interior, Environmental Protection Agency. https://www.epa.gov/eco-research/ecoregions
USDI Geological Survey [USDI USGS]. 2017. Biodiversity Information Serving Our Nation (BISON). U.S. Geological Survey. https://bison.usgs.gov/#home
Utah Crop Improvement Association [UCIA]. 2015. How to be a seed connoisseur. Logan, UT: UCIA, Utah Department of Agriculture and Food, Utah State University and Utah State Seed Laboratory. 16 p.
Utah State University Extension [USU Ext]. 2017. Range plants of Utah, Grays biscuitroot. https://extension.usu.edu/rangeplants/forbsherbaceous/giant-biscuitroot [Accessed 2020 March 5].
Vankus, V. 2006. Association of official seed certifying agencies (AOSCA). https://www.aosca.org/.
Weaver, J.E. 1915. A study of the root systems of prairie plants of southeastern Washington (Concluded). The Plant World. 18(10): 273-292.
Wik, P.A. 2002. Ecology of greater sage-grouse in south-central Owyhee County, Idaho. Moscow, ID: University of Idaho. Thesis. 141 p.
Williams, M.C.; Ralphs, M.H. 1989. Control of Wasatch milkvetch (Astragalus miser var. oblongifolius) and associated species on mountain rangeland. Weed Technology. 3(1): 110-113.
Wolter, B.H.K.; Fonda, R.W. 2002. Gradient analysis of vegetation on the north wall of the Columbia River Gorge, Washington. Northwest Science. 76(1): 61-76.
Young, S.A.; Schrumpf, B.; Amberson, E. 2003. The Association of Official Seed Certifying Agencies (AOSCA) native plant connection. Moline, IL: AOSCA. 9 p. Available: https://seedcert.oregonstate.edu/sites/seedcert.oregonstate.edu/files/pdfs/aoscanativeplantbrochure.pdf
Youssef, N.N.; Bohart, G.E. 1968. The nesting habits and immature stages of Andrena (Thysandrena) candida Smith (Hymenoptera, Apoidea). Journal of the Kansas Entomological Society. 41(4): 442-455.
How to Cite
Gucker, Corey L.; Shaw, Nancy L. 2020. Gray’s biscuitroot (Lomatium grayi). In: Gucker, C.L.; Shaw, N.L., eds. Western forbs: Biology, ecology, and use in restoration. Reno, NV: Great Basin Fire Science Exchange. Online: https://westernforbs.org/species/grays-biscuitroot-lomatium-grayi/