Authorship
Shaw, Nancy; Gucker, Corey
Publication Date
September 2019
Nomenclature
Yellow bee-plant (Peritoma lutea [Hook.] Raf.) is a member of family Cleomaceae, the spiderflower family (Vanderpol and Iltis 2010). Recent molecular work leaves the status of the species and its genus and family in question (e.g., Hall 2008; Iltis et al. 2011; Roalson et al. 2015), but for this review, nomenclature follows the Flora of North America (Vanderpool and Iltis 2010).
Family
Cleomaceae – Spiderflower family
Genus
Peritoma (Cleome)
Species
lutea
NRCS Plant Code
PELU5, CLLU2 (USDA NRCS 2019).
Subtaxa
No subspecies or varieties are recognized by the Flora of North America (Vanderpool and Iltis 2010).
Synonyms
Cleome lutea Hooker, Peritoma breviflora Wooton & Standley (Vanderpool and Iltis 2010).
Common Names
Yellow bee–plant, yellow spiderflower, yellow spiderwort, yellow caper, golden caper, Nevada bee plant (Holmgren and Cronquist 2005; Vanderpool and Iltis 2010; Tilley et al. 2012; Welsh et al. 2016; Calflora 2018; LBJWC 2019; USDA NRCS 2019).
Chromosome Number
2n = 32, 34 (Hitchcock et al. 1964; Holmgren and Cronquist 2005; Vanderpool and Iltis 2010).
Hybridization
No hybrids have been recognized.
Distribution
Yellow bee-plant occurs in arid and semi-arid shrublands east of the Cascade and Sierra Nevada mountains from northern Washington to northwestern New Mexico, southern Arizona, and Baja California, Mexico, and eastward across southern Idaho to western Montana, western Wyoming, and western Colorado (Vanderpool and Iltis 2010; Tilley et al. 2012). It has also been reported from Nebraska (Xerces Society and USDA NRCS 2012; The Jepson Herbarium 2019).
Habitat And Plant Associations
Yellow bee-plant occurs in warm and cold deserts on foothills, plains, mesas, and low mountain valleys. It is best adapted to open, disturbed areas with well-drained soils, ephemerally wet sites, dry washes, sandy flats, and roadsides (Fig. 1) (Iltis 1958; Hitchcock et al. 1964; Holmgren and Cronquist 2005; Vanderpool and Iltis 2010). It grows in full sun and is not shade tolerant (Ogle et al. 2012). Yellow bee-plant occurs in areas receiving 8 to 18 in (200–460 mm) of annual precipitation (USDA NRCS 2011 cited in Tilley et al. 2012).
Populations of yellow bee-plant occur in shadscale (Atriplex confertifolia), sagebrush (Artemisia spp.), horsebrush (Tetradymia spp.), greasewood (Sarcobatus vermiculatus), creosote bush (Larrea tridentata), riparian, pinyon–juniper (Pinus–Juniperus spp.), and ponderosa pine (P. ponderosa) communities (Munz and Keck 1959; Vanderpool and Iltis 2010; Ogle et al. 2012; Tilley et al. 2012; Calflora 2018; Granite Seed 2019). The species spreads quickly from seed and may develop large populations on recent disturbances (Taylor 1992; Cane 2008; Ogle et al. 2012; Granite Seed 2019; LBJWC 2019).
Figure 1. A large population of yellow bee–plant growing in open, disturbed habitat typical for the species. Photo: J. Cane, USDA ARS.
Elevation
Yellow bee-plant grows at elevations from (330) 1,970 to 7,875 ft ([100] 600–2,400 m) (Vanderpool and Iltis 2010). Its elevation range is 3,600 to 5,500 ft (1,100–1,675 m) in California (Munz and Keck 1959), 2,000 to 6,000 ft (610–1,830 m) in Arizona (Kearney and Peebles 1942; Xerces 2012), 4,500 to 6,500 ft (1,370–1,980 m) in New Mexico (Iltis 1958), and 3,000 to 7,810 ft (915– 2,380 m) in Utah (Welsh et al. 2016).
Soils
Plants generally occur on well-drained, medium-textured soils. Yellow bee-plant is most common on sandy soils, but it can be found on moist to dry soils ranging from loamy to rocky (Hitchcock et al. 1964; Anderson and Holmgren 1996; Tilley et al. 2012; LBJWC 2018; Montana Natural Heritage Program 2019; PFAF 2019). It is not adapted to coarse soils (Granite Seed 2019). Yellow bee-plant tolerates pH from 6 to 8 and exhibits moderate tolerance to CaCO3 (Holmgren and Cronquist 2005; USDA NRCS 2011 cited in Tilley et al. 2012; LBJWC 2018).
Description
Yellow bee-plant is a malodorous annual forb that produces a long taproot and one to several erect stems that are simple or with a few branches developing from the upper leaf axils (Holmgren and Cronquist 2005; Vanderpool and Iltis 2010; Welsh et al. 2016).
Stems are glabrous or glabrate to sparsely pilose and 1 to 5 ft (0.3–1.5 m) tall (Hitchcock et al. 1964; Tilley et al. 2012; Welsh et al. 2016). Leaves are alternate, widely spaced (Fig. 2), petiolate, stipulate, palmate, and minutely bristled (setaceous) (Iltis 1958; Turner and Gustafson 2006). Lower leaves may be deciduous. Leaflets are 5 (sometimes 3 or 7), 0.3 to 2 in (0.8 to 5 cm) long, and linear to narrowly elliptic with acuminate tips (Iltis 1958; Vanderpool and Iltis 2010; Welsh et al. 2016).
The showy inflorescences are terminal racemes that produce densely crowded yellow flowers (Iltis 1958; Holmgren and Cronquist 2005). Bracts on the racemes are simple and much smaller than the stem leaves. Flowering is highly indeterminate, beginning at the base of the raceme and proceeding upward as the raceme elongates to about 16 in (40 cm) in fruit (Cane 2008; Vanderpool and Iltis 2010).
Figure 2. Yellow bee-plant growth habit showing the widely separated leaves and brush–like inflorescence. Photo: M. Lavin, Montana State University.
Yellow bee-plant produces perfect and staminate flowers. Perfect flowers produce both stamens and a pistil. Staminate flowers begin as perfect buds, but pistil formation is incomplete, thus flowering is not andromonoecious (with staminate and perfect flowers on the same plant) because truly staminate flowers are not produced (Cane 2008).
Individual flowers are regular or somewhat irregular (Fig. 3) and are borne on ascending pedicels (Holmgren and Cronquist 2005). The four sepals are fused at the base, persistent, lanceolate, acuminate and minutely toothed at the tip (Turner and Gustafson 2006; Vanderpool and Iltis 2010). The corolla is twisted in the bud and consists of four yellow, ovate petals about 0.2–in (6 mm) long. The petals narrow at the base but are not clawed. The ovary of perfect flowers develops on a stalk that is about 0.2 to 0.7 in [5–17 mm] long in fruit (Holmgren and Cronquist 2005; Vanderpool and Iltis 2010; Welsh et al. 2016). Ovaries are large and unilocular with two carpels and parietal placentation. There is a single style (Heywood 1993). The six elongate, yellow stamens are equal and exerted well beyond the petals. The stamens with their conspicuous knobby anthers give the inflorescence a brush-like appearance (Taylor 1992; Holmgren and Cronquist 2005; Turner and Gustafson 2006).
Figure 3. Yellow bee-plant flowers: Perfect flowers with stamens and stalked pistil (left) and staminate flowers (right). Photo: J. Cane, USDA ARS.
The fruit is a pod that is pointed at both ends and suspended and spreading from a curved, 0.2 to 0.5- in (0.6–1.2 cm) long stalk (Iltis 1958; Welsh et al. 2016). Pods are 0.6 to 1.6 in (1.5 to 4.0 cm) long and produce 8 to 20 rugose, ovoid seeds (Cane 2008; Vanderpool and Iltis 2010; Roalson et al. 2015).Seeds are about 0.1 in (2–3 mm) long, gray to black, coiled, and kidney shaped (Cane 2008; Iltis et al. 2011). The testa (outer seed coat) is folded into the curved, cream to white embryo (Iltis et al. 2011). Endosperm may be lacking or very limited (Holmgren and Cronquist 2005).
Iltis (1957) described yellow bee-plant as the primitive form in a reduction series which includes the progressively more specialized genera, Cleomella, Wislizenia, and Oxystylis. He suggested the specializations provided adaptation to progressively more arid environments. Characteristics considered primitive in yellow bee-plant included its large, elongate pods, free-falling seeds, short slender styles, bracteate racemes, small stipules, extensive vegetative growth, and delayed flowering. The presumed specialized genus, Oxystylis, occurs primarily in arid Death Valley.
Reproduction
Yellow bee-plant reproduces entirely from seed. Plants are prolific seed producers in favorable years and can spread rapidly, sometimes establishing large populations on disturbed sites where competition is low (Cane 2008). Annual flowering and seed production vary widely (Cane 2008; S. Jackman, Oregon Wildland Plant Resources [OWPR], personal communication, February 2019).
Phenology
Yellow bee-plant flowers over a period of several weeks beginning in late spring when plants may be only a few inches tall. Flowering continues until late summer or early autumn, depending on location (Munz and Keck 1959; Hitchcock et al. 1964; Taylor 1992; Cane 2008; Vanderpool and Iltis 2010; Welsh et al. 2016). The inflorescences on main stems initiate flowering first, followed by those on the side branches, which develop from the upper leaf axils (Welsh et al. 2016). Flowering begins at the base of the inflorescence and continues upward as the inflorescence elongates (Fig. 4). Thus, older perfect flowers are pollinated, lose their petals, develop pods, and disperse seed while new flowers continue to open near the apex of the elongating inflorescence. Dehiscence of mature fruits occurs when the two halves (valves) of the pod separate from the loop-shaped placenta (replum), which remains on the plant and dehisces separately (Iltis 1958). Seeds then begin dispersing from the placenta (Fig. 5).
Figure 4. An indeterminate yellow bee-plant inflorescence with immature fruits near the base and progressively less mature flowers toward the apex. Photo: M. Lavin, Montana State University.
Racemes alternate between production of perfect and staminate flowers as has also been noted for C. spinosa (spiny spiderflower) (Murneek 1927; Cane 2008). Staminate flower production increases when racemes are producing large numbers of fruits that require high resource input. Thus, flowering continues throughout the season, alternating between the two flower types, and fruit production occurs over a prolonged period (Cane 2008).
Figure 5. Yellow bee-plant flowers, fruits, and seeds. From left to right: perfect flower, immature and mature fruits (pods), fruit with the valves removed leaving the loop-like placenta (replum) and seeds, and placenta with the seeds removed. Photo: J. Cane, USDA ARS.
Breeding System
Cane (2008) found that yellow bee-plant flowers of open-pollinated plants produced two to three times as much fruit as caged plants that received one of three artificial pollination treatments. Caged plants were manually fertilized to compare same flower fertilization (autogamy), fertilization with pollen from a different flower on the same plant (geitonogamy), and cross-pollination (xenogamy). Similar proportions of flowers set fruits in each of the three treatments, indicating that perfect flowers were fully self-fertile. Average counts of viable (dark-colored) seeds (Fig. 6) per silique were comparable across all four treatments (Cane 2008).
Figure 6. Mature, dark, yellow bee-plant seed. Photo: J. Cane, USDA ARS.
In a greenhouse study, seedlings grown from seeds produced by same-plant fertilization treatments were taller and had greater longest leaf lengths than those grown from seed produced by cross-fertilization treatments. This indicated that outcrossing resulted in no seedling vigor advantage (Cane 2008). Self-fertility and greater seedling vigor of seedlings from same-plant pollination (same flower or different flower on the same plant) compared to outcrossing treatments are considered traits favorable for early successional colonists. Self-fertility insures that seeds will be produced, even in areas where plants may be scattered and pollinators scarce or absent following disturbance or where pollinator populations may not be adequate in years of high flower production (Baker and Stebbins 1965; Lloyd 1992; Cane 2008). Yellow bee-plant spread from prolific seed production has been noted in common gardens, and large populations have been observed on disturbed sites (Cane 2008; Tilley et al. 2012).
Pollination
Yellow bee-plant flowers are pollinated by a variety of native generalist bees, wasps, and butterflies that gather nectar and sometimes pollen during the day. Cane (2008) reported that flowers opened 1 to 3 hours after sunset (July 13, Logan, Utah). Stigmas were receptive, and anthers were beginning to dehisce at the distil tip at 2.5 hours after sunset. Nocturnal pollinators were not observed. By dawn, flowers were open, large nectar droplets were present, and the anthers were fully dehisced. Most stigmas (11 of 17 examined), however, had not been pollinated. The remainder were self-pollinated (autogamy), but with 10 grains of pollen or less. By 10:30 am, 14 of 15 stigmas were pollinated, most with more than 15 pollen grains. Thus, some pollination occurred by autogamy at night; this was further enabled as the stamens curled inward later in the day, but diurnal insect pollination was more thorough (Cane 2008). Nocturnal flower opening was considered potentially an ancestral trait as no nocturnal moths or other pollinators were noted. Lack of discernable odor at night and bright flower color would suggest adaptation to diurnal pollination (Faegri and van der Pijl 1979; Dafini et al. 1987; Cane 2008).
The scattered populations of yellow bee-plant may be important food sources for many generalist native bee populations at times when other flowering plants are not abundant (Cane 2008). At the Grand Staircase-Escalante National Monument, yellow bee-plant was considered a magnet species for pollinators, hosting 32 of the 55 bee genera and more than 140 of the 660 bee species identified in the Monument (Cane 2008; Carril et al. 2018). About 25% of the specimens collected were generalist (polylectic) sweat bees (Halictus, Hylaeus) and masked bees (Lasioglossum), while 30% were miner bees (Perdita), which may specialize on other hosts (Cane 2008; Carril et al. 2018).
Because yellow bee-plant populations may produce little or no seed in dry years, the presence of specialist (oligolege) pollinators would not be predicted. However, half of the bee specimens collected on yellow bee-plant in southern Utah were the miner bee, Perdita zebrata, a presumed specialist. Cane (2008) reported that about 90% of the collections of P. zebrata at the USDA Agricultural Research Service Laboratory in Logan, Utah, were made from yellow bee-plant. Most were males, possibly using the flowers as sites for finding mates, a common habit of specialists (Eickwort and Ginsberg 1980).
Ecology
Yellow bee-plant is an early successional colonizer that often grows with few other species on fine- to medium-textured, well–drained soils of disturbed sites in western shrublands (Fig. 7). It spreads readily from seed, grows rapidly, and produces flowers that are both self–fertile and outcrossing. Yellow bee-plant flowers over a long period and hosts a wide array of pollinators, thus supporting pollinator populations for other species (Baker and Stebbins 1965). Flowering, seed production, and population size appear to fluctuate annually, depending upon local precipitation (Cane 2008; S. Jackman, OWPR, personal communication, February 2019). Seedlings may be frost sensitive (PFAF 2019), and plants are apparently not tolerant of competition (Pellett 1940).
Figure 7. Early successional yellow bee-plant growing with few other species. Photo:M. Lavin, Montana State University.
Three Cleome species are known to be C4 species. For this reason, Brown et al. (2005) encouraged development of a seed repository for Cleome species to be used for studies of the genetic basis for the evolution of C4 photosynthesis.
Wildlife And Livestock Use
Yellow bee-plant provides little forage for large mammals and only limited cover for small rodents (Tilley et al. 2012). Pyle (1992) reported use of golden spiderflower (Cleome platycarpa [Peritoma platycarpa]) by greater sage-grouse (Centrocercus urophasianus), but direct use of yellow bee-plant by greater sage–grouse has not been documented.
Insects. Yellow bee-plant is pollinated by a wide variety of bees, wasps, and butterflies (Cane 2008) (see Pollination section). Leaves are used by larvae of Pieris protodice, a cabbage or mustard butterfly (Hovanitz and Chang 1962).
Nutritional Value
Pellett (1940) tested yellow bee-plant as a potential nectar source in his western Iowa gardens near the Missouri River. He considered the species a promising honey plant for the region as it grew rapidly and bloomed from late June until a heavy frost (15°F [–9 °C]) occurred on October 14. Honey bee brood rearing continued longer, and colonies were left in better condition when yellow bee-plant was present. Although the plants did not produce large amounts of pollen, they did secrete abundant nectar, which attracted large numbers of honey bees (Apis mellifera), bumblebees (Bombus spp.), butterflies, and other insects (Pellett 1940). Sugar content of nectar in bees’ honey sacs on September 30, 1939 averaged 23% (range: 15–35%, n=44) (Park cited in Pellett 2940). Establishing the plant in local waste places on the Missouri River bluffs was suggested to provide bee pastures for local honey production (Pellett 1940). Lovell (1968) found the nectar of yellow bee-plant growing with irrigation in Oregon contained only 11.8% sugar (date of the test not provided). He described the honey as dark with a pronounced flavor.
Ethnobotany
The Navajo and Kayenta tribes used yellow bee-plant as a drug or ceremonial medicine and as a dermatological aid for ant bites (Wyman and Harris 1951). Stems and flowers were used by some Plateau tribes to prepare an infusion to treat colds (Hunn 1990).
Horticulture
Yellow bee-plant produces attractive flowers and bright color for several weeks in summer. It can be planted in gardens with medium-textured soils and full exposure to sun. It is recommended for pollinator gardens as it provides resources for many native bees and other insects over its long flowering period (Cane 2008; Cane and Kervin 2013). Yellow bee-plant is particularly valuable for use in naturalizing and beautifying roadways, rest areas, or other low maintenance planting sites that are recovering from disturbance. Plants develop rapidly, tolerate drought, and spread from seed when competition from other species is low. Yellow bee-plant is a commercially available, nectar-rich, low water-use annual recommended for wildflower gardens and urban greenspaces to support monarch butterflies and other pollinators in the Great Basin, Inland Northwest, and Arizona (Fallon et al. 2016a, 2016b; Ley et al. 2019). It has also been grown as a summer annual in Britain (Huxley and Griffiths 1999).
Revegetation Use
Yellow bee-plant is an early successional annual with potential for restoration of disturbed sites in arid and semi-arid areas of the Intermountain West (Cane 2008; Hintz et al. 2016; Ley et al. 2019). It is recommended for planting to improve pollinator habitat in herbaceous wind barriers, field borders, hedgerow plantings, range plantings, and tree and shrub plantings in the Great Basin, Intermountain Semidesert Province, and Arizona (The Xerces Society and USDA NRCS 2012; Fallon et al. 2016 a, b; Ley 2019). Pavek et al. (2013) recommend it for pollinator habitat plantings in the Inland Northwest on sites receiving 9 to 18 in (229–457 mm) of annual precipitation. It also has value for improving aesthetics of low-maintenance landscaping in parks, campgrounds, and along roadways. Seeds germinate readily following overwinter stratification and can produce abundant seedlings in favorable precipitation years. The flowers provide a nectar source for numerous bees, wasps, and butterflies. Because the flowers are self-pollinating and outcrossing, they can produce viable seed on disturbances where pollinators are rare (Cane 2008; Tilley et al. 2012). Although yellow bee-plant is not considered highly competitive, seeding it in selected areas or rows in post-fire seedings on adapted sites could provide a nectar and pollen source for remnant pollinators during the first post-fire season before recovering and seeded forbs begin flowering. Cane (2008) found that 90% of bees collected from yellow bee-plant are ground-nesting species with progeny capable of surviving wildfires in deep underground burrows.
Developing A Seed Supply
For restoration to be successful, the right seed needs to be planted in the right place at the right time. Coordinated planning and cooperation is required among partners to first select appropriate species and seed sources and then properly collect, grow, certify, clean, store, and distribute seed for restoration (PCA 2015).
Developing a seed supply begins with seed collection from native stands. Collection sites are determined by current or projected revegetation requirements and goals. Production of nursery stock requires less seed than large-scale seeding operations, which may require establishment of agricultural seed production fields. Regardless of the size and complexity of any revegetation effort, seed certification is essential for tracking seed origin from collection through use (UCIA 2015).
Seed Sourcing
Yellow bee-plant seed collected from a total of nine populations in four Omernik Level iii Ecoregions (Colorado Plateau, Northern Basin and Range, Central Basin and Range, and Wasatch and Uinta Mountains; Omernik 1987) was germinated and grown in growth chambers to assess differences in early life history and morphological traits that could affect plant establishment and adaptation (Hintz et al. 2016). Differences among populations included proportion of seeds germinated, flowering status, seedling leaf size, and plant height after 3 weeks of exposure to warm conditions (15/9 hr photoperiod at 77/59 °F [24/15 °C]).
Chilling time for germination was correlated with mean temperature of the wettest quarter and annual temperature of the collection site, where populations from colder climates required longer chilling times. Flowering status was significantly related to latitude of the collection site (P = 0.033), thus possibly indicating adaptation to day length or some other latitude–related factor (Hintz 2016). Results suggested that these traits may be adaptive and that seeds should be sourced to match local climatic conditions. Level III Ecoregions did not explain differences among populations. Use of the smaller Provisional Seed Zones (Bower et al. 2014) or Omernik Level iv Ecoregions were considered an alternative for seed sourcing, with research to describe empirical seed zones needed to provide improved sourcing recommendations.
The provisional seed zones identify areas of climatic similarity with comparable winter minimum temperature and aridity (annual heat:moisture index). In Figure 8, Omernik Level III Ecoregions (Omernik 1987) overlay the provisional seeds zones to identify climatically similar but ecologically different areas. For site–specific disturbance regimes and restoration objectives, seed collection locations within a seed zone and ecoregion may be further limited by elevation, soil type, or other factors.
The Western Wildland Environmental Threat Assessment Center’s (USFS WWETAC 2017) Threat and Resource Mapping (TRM) Seed Zone application provides links to interactive mapping features useful for seed collection and deployment planning. The Seedlot Selection Tool (Howe et al. 2017) can also guide restoration planning, seed collection, and seed deployment, particularly when addressing climate change considerations.
Occurrence Map
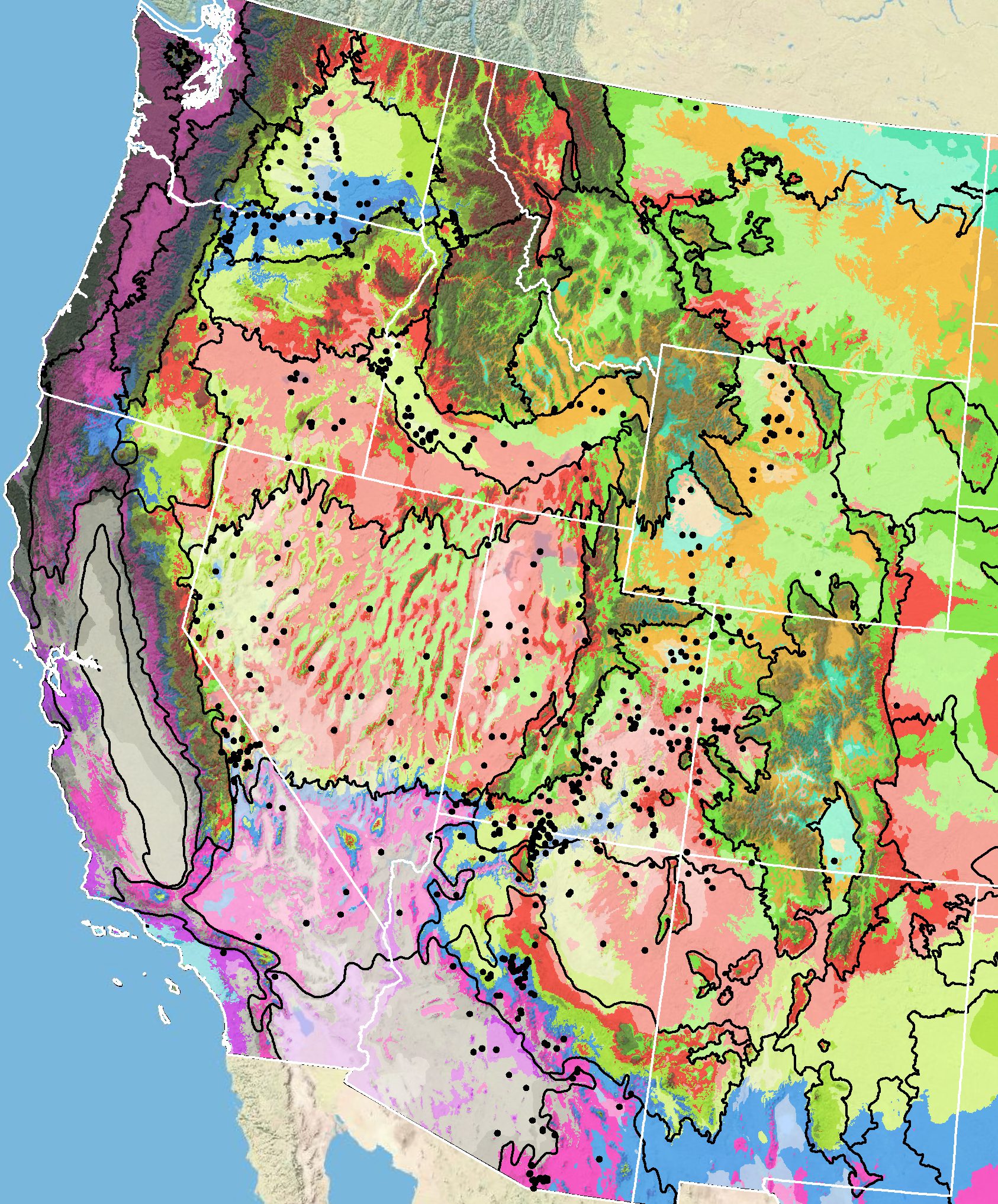
Figure 8. Distribution of yellow bee-plant (black circles) based on geo-referenced herbarium specimens and observational data from 1887-2016 (CPNWH 2017; SEINet 2017; USGS 2017). Generalized provisional seed zones (colored regions; Bower et al. 2014) are overlain by Omernik Level III Ecoregions (black outlines) (Omernik 1987; USDI EPA 2018). Interactive maps, legends, and a mobile app are available (USFS WWETAC 2017; www.fs.fed.us/wwetac/threat-map/TRMSeedZoneMapper2.php?). Map prepared by M. Fisk, USGS.
Releases
As of 2019, there were no yellow bee-plant releases.
Wildland Seed Collection
It is essential to check sites in advance of the expected collection period as seed production and the extent of seed predation by insects is highly variable from year to year (S. Jackman, OWPR, personal communication, February 2019). Prodigious crops may be produced in some years, while practically no seed develops in others.
An x–ray or cut test can be used to provide a check of seed quality as seeds begin to ripen. Cane (2008) reported that seeds that were dark colored at harvest or that darkened within 1 week of harvest contained embryos that were generally viable. Most seeds that remained pale colored generally lacked developed embryos (14 of 20 examined).
Wildland Seed Certification
Verification of species and tracking of geographic source is necessary whether wildland seed is collected for immediate project use or as stock seed for cultivated increase. This official Source Identification process can be accomplished by following procedures established by the Association of Official Seed Certifying Agencies (AOSCA) Pre-Variety Germplasm Program (Young et al. 2020; UCIA 2015). Wildland seed collectors should become acquainted with state certification agency procedures, regulations, and deadlines in the states where they collect.
If wildland–collected seed is to be sold for direct use in ecological restoration projects, collectors must apply for Source-Identified certification prior to making collections. Pre-collection applications, site inspections, and species and seed amount verification are handled by the AOSCA member state agency where seed collections will be made (see listings at AOSCA.org).
If wildland seed collected by a grower or private collector is to be used as stock seed for planting cultivated seed fields or for nursery propagation (See Agricultural Seed Field Certification section), detailed information regarding collection site and collecting procedures must be provided when applying for certification. Photos and herbarium specimens may be required. Germplasm accessions acquired within established protocols of recognized public agencies, however, are normally eligible to enter the certification process as stock seed without routine certification agency site inspections. For contract grow–outs, however, this collection site information must be provided to the grower to enable certification.
Collection Timing
There is generally a wide collection window for harvesting seed as plants ripen indeterminately over a long period from May to October depending on location. Collections may be made on multiple dates if sites are easily accessible. In addition, and depending upon site topography and conditions, plants in different microsites within an area (depressions, ridges, slopes, etc.) may be at different flowering and fruit ripening stages on any one collection date.
For 30 collections made by USDI, Bureau of Land Management, Seeds of Success (SOS) personnel, harvest dates ranged May 30, 2004, in Wayne County, Utah, to October 30, 2010, in Churchill County, Nevada (USDI BLM SOS 2017). Collections ranged in elevation from 2,223 ft (678 m) in Malheur County, Oregon, collected on July 12, 2009 to a highest elevation of 7,183 ft (2,189 m) in Wayne County, Utah, collected on September 22, 2009.
Collection Methods
Fruit ripening is highly indeterminate with fruit at the base of the raceme ripening earliest. At maturity, the two valves of mature pods begin separating from the loop-like placenta (replum) (Fig. 5). Seeds are attached to the placenta, but soon begin dispersing. Collectable fruits are those that dehisce readily or that have begun to dehisce along the valves, leaving the seeds visible (S. Jackman, OWPR, personal communication, February 2019). These can be harvested with minimal disturbance to immature fruits further up the raceme. Fruits can be stripped by hand or using a flat beater (e.g., tennis racket) into bags, boxes, or a collection hopper. Thin gloves may be worn to protect the hands from abrasion. Collections will consist of a mixture of seeds, valves, and fine debris. Although wildland populations are often quite dense, they are generally not pure enough or extensive enough to warrant mechanical harvesting (S. Young, Utah State University [emeritus], personal communication, February 2019).
Several collection guidelines and methods should be followed to maximize the genetic diversity of wildland collections: 1) collect seed from a minimum of 50 randomly selected plants; 2) collect from widely separated individuals throughout a population without favoring the most robust or avoiding small stature plants; and 3) collect from all microsites including habitat edges (Basey et al. 2015). General collecting recommendations and guidelines are provided in online manuals (e.g., ENSCONET 2009; USDI BLM SOS 2021).
It is critical that wildland seed collection does not impact the sustainability of native plant populations. Collectors should take no more than 20% of the viable seed available at the time of harvest (USDI BLM SOS 2021). Additionally, care must be taken to avoid the inadvertent collection of weedy species, particularly those that produce seeds similar in shape and size to those of yellow bee-plant.
Post-Collection Management
Collections should be dried thoroughly as some pods and vegetative material in the collection may not be completely dry at the time of harvest. The seed lot should be spread over screens in a thin layer to provide for good air circulation. Seeds should be dried in a location free of rodents and treated to control insects as necessary with the use of appropriate insecticides or by freezing once the seed is dry.
Seed Cleaning
Seeds are easily cleaned to high levels of purity (Fig. 9). At the USFS Lucky Peak Nursery, seed lots are first run over a scalper with top screen no. 8 and bottom screen no. 1/16. Screen sizes may require some adjustment for individual seed lots. Fine cleaning is accomplished using a Missoula dewinger (USFS Missoula Technology and Development Center, Missoula, MT) with feeder speed 4, drum speed 6, angle 8, and the air closed to remove fine chaff and non–viable seed (usually light colored) (USFS LPN 2017). At the USFS Bend Seed Extractory, large stems are removed by hand. Seed is then sieved from the seed lot using soil sieve screens, usually numbers 4, 5, or 6 to remove the pod valves and other coarse material. A Westrup LA–P de-awner (Westrup A/S, Slagelse, Denmark) is then used to break up remaining pod material and release any seed that remains in the pods. The seed lot is then sieved again to remove free seeds. If any seed remains in the pod material at that point, another cycle of de-awning and sieving is conducted. After each step, freed seeds are removed to reduce the risk of mechanical damage by repeated abrasion as well as the quantity of material yet to be cleaned (K. Herriman, USFS Bend Seed Extractory, personal communication, March 2019).
Figure 9. Clean, primarily dark colored yellow bee-plant seed. Photo: USFS.
Seed Storage
Seeds are orthodox and should be stored dry. Literature on seed longevity and storage requirements is lacking.
Seed Testing
There is no AOSA rule for testing the germination of Peritoma species (AOSA 2016). Purity tests can be conducted using standard procedures. Viability is tested as it would be for other Cleomaceae (AOSA 2010). A thin slice is cut along the widest dimension of imbibed seed. Seeds are then stained in 0.1% tetrazolium chloride (TZ) overnight. Viable normal embryos are those that stain evenly; slight damage to the radicle is acceptable.
Viability Testing
Cane (2008) found that dark–colored seed and seed that turned dark within 1 week of harvest contained fully developed, viable embryos and were readily germinable following cool stratification. Light-colored seeds with pale embryos in x–rays generally lacked fully developed embryos (14 of 20 tested).
Germination Biology
Laboratory prechilling or fall planting to provide overwinter exposure to prechilling temperatures is required to relieve seed dormancy and enhance germination (Tilley et al. 2012; Foxx and Kramer 2015; J. Cane, USDA ARS, personal communication, March 2019). Viable seeds from the Colorado Plateau area of Uinta County, Utah, germinated to 75% or more when subjected to treatments that included exposure of imbibed seeds to 12 weeks at 34 °F (1 °C ) (simulating winter temperatures in this area) followed by incubation for 4 weeks at one of three alternating day/night (12/12 hrs) spring temperatures: 1) early spring (52/34 °F [11/1 °C]), 2) mid-spring (59/41 °F [15/5 °C]), or 3) late spring (68/50 °F [20/10 °C). Germination without the overwinter treatment and exposure to 4 weeks of any of the three spring conditions resulted in 5% germination or less (Foxx and Kramer 2015). Seeds were incubated in growth chambers on 1.5% agar. As an alternative to prechilling, partial seed coat removal and incubation on 1% agar with 250 mg/l gibberellic acid (GA3) followed by incubation at 73/48 °F (23/9 °C) (12 hrs light/12 hrs dark) for 42 days resulted in 78% germination (RBG Kew 2017).
Hintz et al. (2016) compared early life history traits of nine yellow bee-plant accessions from four Omernik Level iii Ecoregions in the Interior West (Northern and Central Great Basin, Colorado Plateau and the Wasatch and Uinta Mountains) in a growth chamber experiment. Chilling time required for germination was positively correlated with mean temperature of the wettest quarter and mean annual temperature at the collection site. Sites with higher mean annual temperatures had greater percent germination after 25 days in cold stratification (P = 0.008).
Wildland Seed Yield And Quality
Post–cleaning seed yield and quality of seed lots collected in the Intermountain region are provided in Table 1 (USFS BSE 2017). The results indicate that yellow bee-plant can generally be cleaned to high levels of purity and seed fill and that viability of fresh seed is generally high.
Seeds cleaned at the USFS Bend Seed Extractory averaged 89,704 PLS/lb (197,763/kg). High variability in clean-out ratios may have resulted from differences in the quantity of pod pieces collected in the bulk seed lot. The number of seeds per pound varied considerably among lots, even though most seeds in the cleaned lots were viable (Table 1; USFS BSE 2017). Other sources report a greater range of 68,040 to 222,131 seeds/lb (150,003 to 489,715 seeds/kg) of cleaned seed (Cane 2008; USFS GBNPP 2014; RBG Kew 2017; Shock et al. 2017a; Granite Seed 2019; Stevenson Intermountain Seed 2019).
Table 1. Seed yield and quality of yellow bee-plant seed lots collected in the Intermountain region, cleaned by the Bend Seed Extractory, and tested by the Oregon State Seed Laboratory or the USFS National Seed Laboratory (USFS BSE 2017).
Seed lot characteristic |
Mean |
Range |
Samples (no.) |
Bulk weight (lbs) |
1.34 |
0.09–11.5 |
21 |
Clean weight (lbs) |
0.65 |
0.012–5.88 |
21 |
Clean–out ratio |
0.48 |
0.02–0.91 |
21 |
Purity (%) |
99 |
97–99 |
21 |
Fill (%)¹ |
94 |
77–99 |
21 |
Viability (%)² |
94 |
89–98 |
19 |
Seeds/lb |
95,873 |
63,000–121,000 |
21 |
Pure live seeds/lb |
89,704 |
56,620–110,327 |
19 |
¹ 100 seed X–ray test
² Tetrazolium chloride test
Marketing Standards
Yellow bee-plant seed is often commercially available (Tilley et al. 2012), but quantities may be small (Xerces Society and USDA NRCS 2012). No quality standards have been published. Acceptable seed purity, viability, and germination specifications vary with revegetation plans. Purity needs are highest for precision seeding equipment like that used in nurseries, while some rangeland seeding equipment handles less clean seed quite well.
Agricultural Seed Production
Much of the information regarding culture of yellow bee-plant for seed production, comes from test plots grown at Oregon State University’s Malheur Experiment Station (OSU MES) (Shock et al. 2017b, 2018, 2019).
Agricultural Seed Certification
In order to minimize genetic changes in specific accessions of native species when increased in cultivated fields, it is essential to track the geographic source and prevent inadvertent hybridization or selection pressure. This is accomplished by following third party seed certification protocols for Pre–Variety Germplasm (PVG) as established by the Association of Official Seed Certification Agencies (AOSCA). AOSCA members in the U.S., Canada, and other countries administer PVG requirements and standards that track the source and generation of planting stock. Field and cleaning facility inspections then monitor stand establishment, proper isolation distances, control of prohibited weeds, seed harvesting, cleaning, sampling, testing, and labeling for commercial sales (Young et al. 2020; UCIA 2015).
Seed growers apply for certification of their production fields prior to planting and plant only certified stock seed of an allowed generation (usually less than four). The systematic and sequential tracking through the certification process requires preplanning, knowing state regulations and deadlines, and is most smoothly navigated by working closely with state certification agency personnel. See the Wildland Seed Certification section for more information on stock seed sourcing.
Site Preparation
Yellow bee-plant should be fall seeded with a drill or by broadcast seeding in a weed-free seedbed. Overwinter incubation in moist soil permits imbibition and overwinter prechilling to relieve physiological seed dormancy (Tilley et al. 2012). Dry soil conditions in winter or spring preclude exposure to adequate prechilling to release dormancy (Tilley et al. 2012; J. Cane, USDA ARS, personal communication, March 2019). Tilley et al. (2012) recommended that seed be planted at a rate of 10 lbs PLS/ac (11 kg/ha) and at a depth of 0.1 to 0.25 in (3–6 mm). Seeding yellow bee-plant at a depth of 0.25 to 0.5 in (0.6 to 1.3 cm) at a rate of 14 lbs PLS/ac (15.7 kg/ha) in fine to medium soils was recommended by Pavek et al. (2013).
At OSU MES near Ontario, Oregon, yellow bee-plant was seeded in beds with rows spaced 30 in (76 cm) apart on Nyssa silt loam soil (pH 8.3, 1.1% organic matter) for irrigation research studies (Shock et al. 2017b, 2018, 2019). Seeding was done annually in mid-November from 2011 through 2017 using a small-plot grain drill with disc openers (Shock et al. 2017b, 2018, 2019). Seed was planted on the soil surface at the rate of 20 to 30 seeds/linear ft (66–98/m). Sawdust was then applied in a narrow band over each seed row at a rate of 0.26 oz/ft of row (24 g/m). The beds were then covered with row cover (N–sulate, DeWitt Co., Inc., Sikeston, MO) using a mechanical plastic mulch layer. The row cover was removed in April following seedling emergence. Beginning in 2013, netting was placed over the plots to prevent predation by birds (Shock et al. 2017).
Weed Management
Shock et al. (2017a) treated yellow bee-plant stand establishment research plots at OSU MES with Sethoxydim on April 6, 2016, immediately after removal of row cover. Effectiveness was not provided. Plots were hand weeded thereafter. Plots established for irrigation studies at the OSU MES were hand weeded (Shock et al. 2017b, 2018, 2019).
Establishment And Growth
Stands may be erratic, even with fall planting (Shock et al. 2019). Developing seedlings are vulnerable to predation by birds. Seedlings grow rapidly and may begin flowering when only a few inches tall. Flowering and seed production extends over a long period, and inflorescences continue to elongate during the flowering period (Munz and Keck 1959; Hitchcock et al. 1964; Taylor 1992; Cane 2008; Vanderpool and Iltis 2010; Welsh et al. 2016).
Irrigation
At OSU MES, irrigation research plots of yellow bee-plant were seeded each fall from 2011 to 2017 (Shock et al. 2019). Seeds were planted in strips with four rows, 30 inches (76 cm) apart on Nyssa silt loam (pH 8.3, 1.1% organic matter). Subsurface drip irrigation was used to minimize surface wetting, weed establishment, and the risk of damage resulting from infection by fungal pathogens. Established plots received 0, 4 or 8 in (0, 100, or 200 mm) of subsurface drip irrigation in four equal applications about 2 weeks apart between floral bud formation and seed set (Table 2). In 2014, three additional irrigations were applied beginning on August 12 to determine whether the flowering and seed production period could be extended (Shock et al. 2019).
Seed yield was highest without irrigation in 2016 (Table 3). Yields did not respond to irrigation in the other years tested (2012, 2014, 2015, and 2018). Stands were lost to flea beetles in 2013 and to poor emergence in 2017. Additional irrigations in 2014 did not result in an extension or resumption of flowering (Shock et al. 2019).
Table 2. Yellow bee-plant flowering, irrigation, and seed harvest dates at the Oregon State University, Malheur Experiment Station, Ontario, Oregon, 2012 to 2018 (Shock et al. 2019).
Year* |
Flowering date |
Irrigation date |
Harvest date |
|||
Start |
Peak |
End |
Start |
End |
||
2012 |
May 16 |
Jun 15 |
Jul 30 |
May |
Jun 13 |
Jul 12– |
2014 |
Apr 29 |
Jun 4 |
Jul 22 |
Apr |
Jun 3 |
Jun 23– |
2015 |
Apr 8 |
May 13 |
Jul 6 |
Apr |
May 27 |
Jun 4– |
2016 |
Apr 13 |
May 13 |
Jul 25 |
Apr |
May 31 |
Jun 14– |
2018 |
Apr 23 |
Jun 13 |
Aug 20 |
May |
Jun 13 |
Jun 1– |
*Full stand loss to flea beetles in 2013, poor stand emergence in 2017.
Table 3. Yellow bee-plant seed yield response to irrigation at the Oregon State University, Malheur Experiment Station, Ontario, Oregon, 2012 to 2018. Within years, seed yields followed by different letters differed significanlty (P < 0.05) (Shock et al. 2019).
Year ¹
|
Seed yield (lbs/ac) with supplemental irrigation² |
||
0 in |
4 in |
8 in |
|
2012 |
99.7 |
74.7 |
99.4 |
2014 |
184.8 |
197.8 |
162.1 |
2015 |
122.2 |
71.8 |
100.8 |
2016 |
58.5b |
43.6a |
31.2a |
2018 |
1.2 |
0.5 |
0.6 |
Average |
93.3 |
77.7 |
78.9 |
¹ Full stand loss to flea beetles in 2013, poor stand emergence in 2017.
² Irrigation season from floral bud to seed set.
Figure 10. Yellow bee-plant irrigation trial at the Oregon State University Malheur Experiment Station, Ontario, Oregon. Photo: N. Shaw, USFS.
Pollinator Management
Yellow bee-plant flowers are self-fertile, but Cane (2008) found two to three times as many fruits developed when pollinators were present and cross pollination occurred. Although yellow bee-plant hosts a wide number of pollinators (Cane 2008; Carril et al. 2018), generalist domestic bees (European honey bees [Apis mellifera] and alfalfa leaf-cutting bees [Megachile rotundata]) can be used to pollinate seed fields. Because of the huge numbers of flowers produced by yellow bee-plant, adequate numbers of pollinators must be available to provide cross pollination and insure good seed crops. Cane (2008) recommended providing habitat on farms for native ground nesting bees that cannot be managed as yellow bee-plant would serve as a host to many of these species on farm. As their populations developed these bees could provide pollinator services to other native species being grown.
Pest Management
Tilley et al. (2012) reported extensive damage to yellow bee-plant foliage caused by western cabbage flea beetles (Phylotreta cruciferae) at the USDA Natural Resource Conservation Service’s Aberdeen Plant Material Center near Aberdeen, Idaho. The beetles multiply on the mature plants, and larvae may move to the roots, overwintering as pupae (J. Cane, USDA ARS, personal communication, March 2019). At OSU MES, Shock et al. (2017) found that early attention to flea beetles was essential for yellow bee-plant seed production to preclude substantial foliage damage. In 2012 plants in a seed production research plot were sprayed in April and June to control flea beetles. Infestations caused complete stand loss of emerging seedlings from 2012 fall-seeded yellow bee-plant in spring 2013. Beetles were not observed in 2014. Following March removal of row cover from fall seeded plots in 2015 through 2018, flea beetle damage was noted, and plots were treated with insecticides in March or April. In 2017, stands were treated on five dates from April to early August to control flea beetle infestations (Shock et al. 2017a, b; 2018; 2019).
Farr and Rossman (2018) report that the following fungi utilize yellow bee-plant as a host: Cercospora conspicua (Pseudocercospora conspicua), Piccinia aristidae, and P. subnitens (Aecidium biforme). Tilley et al. (2012) observed powdery mildew (Leveillula taurica) on yellow bee-plant.
Seed Harvesting
Seed can be harvested using a vacuum harvester or by swathing and allowing the swathed material to dry for a week before harvesting the seed (Tilley et al. 2012). At OSU MES, research plots were harvested manually two to four times each year (Shock et al. 2017b). Multiple non-destructive harvests increased total seed yield and its genetic diversity by harvesting seed from all stages of flowering.
Seed Yields And Stand Life
Cane (2008) found that each mature, open-pollinated plant in a common garden produced an average of 11,000 seeds. He estimated that a seed field could produce about 4,400 lbs/ac (2,000 kg/ha), though this would require multiple harvests. Because of indeterminate ripening, some seed is usually lost. Variation in seed yield also results from poor establishment, poor seed production– possibly related to low precipitation, flea beetle infestations, and harvesting method and date(s). Over a 7-year period at OSU MES, yields were not improved with 4 or 8 in (10–20 cm) of irrigation (Table 3; Shock et al. 2019).
Nursery Practice
Seeds require prechilling for greenhouse production (See the Germination biology section). Seed can be prechilled in moist peat or sand at 39 to 41 °F (4–5 °C) for 30 to 190 days (Tilley et al. 2012). Germination of adequately prechilled seed will occur in 5 to 14 days if seed is incubated at 77 °F (25 °C) in a greenhouse. Germination may be depressed by daytime temperatures below 68 °F (20 °C) (Tilley et al. 2012; PFAF 2019). Germinants can be moved to containers and grown in a greenhouse.
Wildland Seeding And Planting
Yellow bee-plant is a useful colonizer for burned or otherwise disturbed sites where adapted. It is capable of self-pollinating where pollinators are limited or lacking and spreads readily from seed where competition is low (Cane 2008; Tilley et al. 2012). It is an important forage species for pollinators including many species of bees, wasps, and butterflies. As a prolifically flowering annual with a long flowering season, its inclusion in seeding mixes could provide food for insects during the first post–fire season. This would sustain pollinators until seeded perennial forbs begin flowering, usually not until the second post-seeding season (Cane 2008; Ogle et al. 2011).
Yellow bee-plant seed may be most efficiently used if seeded in a weed-free seedbed in patches or rows separate from other species or with other disturbance-adapted species that are not highly competitive. It should be fall seeded by drilling or broadcasting to provide overwinter stratification (Tilley et al. 2012). Dry soil through the winter may preclude stratification and dormancy release. Seeding depths of 0.1 to 0.2 in (3–6 mm) (Ogle et al. 2011; Tilley et al. 2012) or 0.25 to 0.5 in (6–13 mm) are recommended (Pavek et al. 2013; Stevenson Intermountain Seed 2019); deeper seed placement should be avoided. Yellow bee-plant can be seeded at rates ranging from 7 to 14 PLS lbs/ac (8–16 kg/ha) to obtain a full stand (Tilley et al. 2012; Pavek et al. 2013; Granite Seed 2019). Rates should be adjusted for wildland plantings depending on composition of the seed mix. Seed may be drilled in seed fields from selected drill drops or in patches with compatible species.
Acknowledgements
Funding for Western forbs: Biology, Ecology, and Use in Restoration was provided by the USDI BLM Great Basin Native Plant Materials Ecoregional Program through the Great Basin Fire Science Exchange. We thank chapter reviewers Matthew Fisk, US Geological Survey, and Therese Meyer, Utah Department of Natural Resources, Division of Wildlife Resources.
This research was supported in part by the USDA Forest Service, Rocky Mountain Research Station. The findings and conclusions in this publication are those of the authors and should not be construed to represent any official USDA or U.S. Government determination or policy.
Literature Cited
Andersen, B.A.; Holmgren, A.H. 1996. Mountain plants of northeastern Utah. Logan, UT: Utah State University, Extension Services. 148 p.
Association of Official Seed Analysts [AOSA]. 2010. AOSA/SCST Tetrazolium testing handbook. Contribution No. 29. Lincoln, NE: Association of Official Seed Analysts.
Association of Official Seed Analysts [AOSA]. 2016. AOSA rules for testing seeds. Vol. 1. Principles and procedures. Washington, DC: Association of Official Seed Analysts.
Association of Official Seed Certifying Agencies [AOSCA]. 2003. The AOSCA native plant connection. Moline, IL: Association of Official Seed Certifying Agencies. 8 p.
Baker, H.G.; Stebbins, G.L. 1965. The genetics of colonizing species. New York, NY: Academic press. 588 p.
Basey, A.C.; Fant, J.B.; Kramer, A.T. 2015. Producing native plant materials for restoration: 10 rules to collect and maintain genetic diversity. Native Plants Journal. 16(1): 37-53.
Bower, A.D.; St. Clair, J.B.; Erickson, V. 2014. Generalized provisional seed zones for native plants. Ecological Applications. 24(5): 913-919.
Brown, N.; Parsley, K.; Hibberd, J.M. 2005. The future of C4 research – Maize, Flaveria or Cleome? TRENDS in Plant Science. 10(5): 215-221.
Calflora. 2018. Cleome lutea. 2018. Berkeley, CA: The Calflora Database. http://www.calflora.org [Accessed 2018 April 4].
Cane, J.H. 2008. Beeding biologies, seed production and species-rich bee guilds of Cleome lutea and Cleome serrulata (Cleomaceae). Plant Species Biology. 23(3): 152-158.
Cane, J.H.; Kervin, L. 2013. Gardening for native bees in Utah and beyond. ENT-133-09. Logan,UT: Utah State University Cooperative Extension and Utah Plant Pest Diagnostic Laboratory. 13 p.
Carril, O.M.; Griswold, T.; Haefner, J.; Wilson, J.S. 2018. Wild bees of Grand Staircase-Escalante National Monument; richness, abundance, and spatio-temporal beta-diversity. PeerJ 6:e5867. 29 p.
Consortium of Pacific Northwest Herbaria [CPNWH]. 2017. Seattle, WA: University of Washington Herbarium, Burke Museum of Natural History and Culture. http://www.pnwherbaria.org/index.php
Dafni, A.; Eisikowitch, D.; Ivri, Y. 1987. Nectar flow and pollinators efficiency in two co-occurring species of Capparis (Capparaceae) in Israel. Plant Systematics and Evolution. 157(3-4): 181-186.
Eickwort, G.C.; Ginsberg, H.S. 1980. Foraging and mating behavior in Apoidea. Annual Review of Entomology. 25: 421-446.
European Native Seed Conservation Network [ENSCONET]. 2009. ENSCONET seed collecting manual for wild species. Edition 1: 32 p.
Faegri, K.; van der Pijl, L. 1979. The principles of pollination ecology. New York, NY: Pergamon Press. 304 p.
Fallon, C.; Adamson, N.L.; Jepsen, S.; Sardinas, H.; Stine, A.; Vaughan, M. 2016. Monarch nectar plants: Great Basin. Portland, OR: The Xerces Society for Invertebrate Conservation. 3 p.
Farr, D.F.; Rossman, A.Y. 2017. Fungal databases, U.S. National Fungus Collections. U.S. Department of Agriculture, Agricultural Research Service. https://nt.ars-grin.gov/fungaldatabases/.
Foxx, A.; Kramer, A. 2015. Propagation protocol for production of propagules (seeds, cuttings, poles, etc.) Cleome lutea Hook. seeds. Chicago Botanic Garden – Research Glencoe, Illinois. Native Plant Network. U.S. Department of Agriculture, Forest Service, National Center for Reforestation, Nurseries, and Genetic Resources. http://NativePlant Network.org [Accessed 2019 March 3].
Granite Seed. 2019. Cleome lutea beeplant or yellow spiderflower. https://graniteseed.com/products/cleome-lutea [Accessed 2019 March 21].
Hall, J.C. 2008. Systematics of Capparaceae and Cleomaceae: An evaluation of the generic delimitations of Capparis and Cleome using plastid DNA sequence data. Botany. 86(7): 682-696.
Heywood, V.H. 1993. Flowering plants of the world. Oxford, UK: Oxford University Press. 335 p.
Hintz, L.; Eshleman, M.M.; Foxx, A.; Wood, T.E.; Kramer, A. 2016. Population differentiation in early life history traits of Cleome lutea var. lutea in the Intermountain West. Western North American Naturalist. 76(1): 6-17.
Hitchcock, C.L; Cronquist, A.; Ownbey, M.; Thompson, J.W. 1964. Part 2: Salicaceae to Saxifragaceae. Vascular plants of the Pacific Northwest. Seattle, WA: University of Washington Press: 597 p.
Holmgren, P.K.; Cronquist, A. 2005. Cleomaceae, the cleome or spider-flower family. In: Holmgren, N.H.; Holmgren, P.K.; Cronquist, A. Intermountain flora: Vascular plants of the Intermountain West, U.S.A. Volume Two, Part B: Subclass Dilleniidae. Bronx, NY: New York Botanical Garden Press: 160-166.
Hovanitz, W.; Chang, V.C.S. 1962. The effect of various food plants on survival and growth rate of Pieris. Journal of Research on the Lepidoptera. 1(1): 21-42.
Howe, G.; St, Clair, B.; Bachelet, D. 2017. Seedlot Selection Tool. Corvallis, OR: Conservation Biology Institute. https://seedlotselectiontool.org/sst/
Hunn E.S. 1990. NchiWana. The big river: Mid-Columbia Indians and their land. Seattle: University of Washington Press. 384 p.
Huxley, A.; Griffiths, M., eds. 1999. The new RHS dictionary of gardening. 1992. London, UK: MacMillan Press. 3000 p.
Iltis, H.H. 1957. Studies in the Capparidaceae. III. Evolution and phylogeny of the western North American Cleomoideae. Annals of the Missouri Botanic Garden. 44(1): 77-119.
Iltis, H.H. 1958. Studies in the Capparidaceae: V. Capparidaceae of New Mexico. The Southwestern Naturalist. 3(1/4): 133-144.
Iltis, H.H.; Hall, J.C.; Cochrane, T.S.; Sytsma, K.J. 2011. Studies in the Cleomaceae I. On the separate recognition of Capparaceae, Cleomaceae, and Brassicaceae. Annals of the Missouri Botanical Garden. 98(1): 28-36.
Kearney, T.H.; Peebles, R.H. 1942. Flowering plants and ferns of Arizona. Misc. Pub. 423. Washington, DC: U.S. Department of Agriculture. 1069 p.
Lady Bird Johnson Wildflower Center [LBJWC]. 2019. Cleome lutea Hook. Native Plant Database. Austin, TX: Lady Bird Johnson Wildflower Center. https://www.wildflower.org/plants-main [Accessed 202 March 21].
Ley, E.L.; Buchmann, S.; Stritch, L.; Soltz, G. 2019. Selecting plants for pollinators: A regional guide for farmers, land managers and gardeners in the ecological region of the Intermountain Semidesert Province including states of Washington, Oregon, Idaho, Wyoming and parts of California, Nevada, Utah, Montana, Colorado. San Francisco, CA: The Pollinator Partnership/North American Pollinator Protection Campaign. 23 p. https://www.pollinator.org/PDFs/Intermt.SemiDsrt.Desert.rx2.pdf [Accessed 2019 March 27].
Lloyd, D.G. 1992. Self- and cross-fertilization in plants. II. The selection of self-fertilization. International Journal of Plant Sciences. 153(3): 370-380.
Lovell, H.B. 1968. Lets talk about honey plants. Gleanings in Bee Culture. 96(11): 675-676, 697.
Montana Natural Heritage Program. 2019. Yellow beeplant-Cleome lutea. http://fieldguide.mt.gov/speciesDetail.aspx?elcode=PDCPP03070&sort=1 [Accessed 2019 March 21].
Munz, P.A.; Keck, D.D. 1973. A California flora and supplement. Berkeley, CA: University of California Press. 1905 p.
Murneek, A.E. 1927. Physiology of reproduction in horticultural plants. II. The physiological basis of intermittent sterility with special reference to the spider flower. Columbia, MO: University of Missouri Agricultural Experiment Station Research Bulletin. 106: 1-37.
Ogle, D.; St. John, L.; Stannard, M.; Holzworth, L. 2012. Conservation plant species for the Intermountain West. Plant Materials Technical Note 24. Boise, ID: U.S. Department of Agriculture, Natural Resources Conservation Service. 57 p.
Ogle, D.; Tilley, D.; Cane, J.; St. John, L.; Fullen, K.; Stannard, M.; Pavek, P. 2011. Plants for pollinators in the Intermountain West. Plant Materials Tech. Note 2A. Boise, ID: U.S. Department of Agriculture, Natural Resources Conservation Service. 40 p.
Omernik, J.M. 1987. Ecoregions of the conterminous United States. Map (scale 1:7,500,000). Annals of the Association of American Geographers. 77(1): 118-125.
Pavek, P.; Fleenor, R.; Stannard, M.; Dring, T.; Cane, J.; St. John, L.; Tilley, D. 2013. Plants for pollinators in the Inland Northwest (revised). Boise, ID: U.S. Department of Agriculture, Natural Resources Conservation Service, Pullman Plant Materials Center. 62 p.
Pellett, F.C. 1940. From our honey plant test gardens. American Bee Journal. 1940(January): 16-17.
Plants for a future [PFAF]. 2019. Cleome lutea-Hook. https://pfaf.org/user/Plant.aspx?LatinName=Cleome+lutea [Accessed 2019 March 21].
Pyle, W.H. 1992. Response of brood-rearing habitat of sage grouse to prescribed burning in Oregon. Corvallis, OR: Oregon State University. Thesis. 47 p.
Roalson, E.H.; Hall, J.C.; Riser, J.P.; Cardinal-McTeague, W.M.; Cochrane, T.S.; Sytsma, K.J. 2015. A revision of generic boundaries and nomenclature in the North American cleomoid clade (Cleomaceae). Phytotaxa. 205(3): 129-144.
Royal Botanic Gardens Kew [RBG Kew]. 2017. Seed Information Database (SID). Version 7.1. http://data.kew.org/sid/
SEINet – Regional Networks of North American Herbaria Steering Committee [SEINet]. 2017. SEINet Regional Networks of North American Herbaria. https://symbiota.org/seinet
Shock, C.C.; Feibert, E.B.G.; Rivera, A.; Sanders, L.D.; Kilkenny, F.; Shaw, N. 2017a. Direct surface seeding systems for the establishment of native plants in 2016. In: Shock, C.C., ed. Malheur Experiment Station Annual Report 2016. OSU AES Ext/CRS 157. Corvallis, OR: Oregon State University: 123-130.
Shock, C.C.; Feibert, E.B.G.; Rivera, A.; Sanders, L.D.; Shaw, N.; Kilkenny, F. 2017b. Native beeplant seed production in response to irrigation in a semi-arid environment. In: Shock, C.C., ed. Malheur Experiment Station Annual Report 2016. OSU AES Ext/CRS 157. Corvallis, OR: Oregon State University: 140-144.
Shock, C.C.; Feibert, E.B.G.; Rivera, A.; Sanders, L.D.; Shaw, N.; Kilkenny, F. 2018. Native beeplant seed production in response to irrigation in a semi-arid environment. In: Shock, C.C., ed. Malheur Experiment Station Annual Report 2017. OSU AES Ext/CRS 159. Corvallis, OR: Oregon State University: 154-159.
Shock, C.C.; Feibert, E.B.G.; Rivera, A.; Wieland, K.; Shaw, N.; Kilkenny, F. 2019. Native beeplant seed production in response to irrigation in a semi-arid environment. In: Shock, C.C., ed. Malheur Experiment Station Annual Report 2018. OSU AES Ext/CRS 161. Corvallis, OR: Oregon State University. 7 p.
Stevenson Intermountain Seed, Inc. 2019. Cleome lutea yellow beeplant (Native). Ephraim, UT: Stevenson Intermountain Seed, Inc. http://stevensonintermountainseed.com/shop/cleome-lutea/ [Accessed 2019 March 28].
Stromberg, J.C. 2013. Root patterns and hydrogeomorphic niches of riparian plants in the American Southwest. Journal of Arid Environments. 94: 1-9.
Taylor, R.J. 1992. Sagebrush country: A wildflower sanctuary. Missoula, MT: Mountain Press Publishing Company. 211 p.
The Jepson Herbarium. 2019. Peritoma lutea yellow bee plant. Berkeley, CA: University of California – Berkeley. http://ucjeps.berkeley.edu/eflora/eflora_display.php?tid=91909 [Accessed 2019 March 21].
The Xerces Society and USDA NRCS. 2012. Plants for enhancing pollinator habitat in Arizona. Tech. Notes TN-Plant Materials-12-1-Arizona. The Xerces Society for Invertebrate Conservation and USDA Natural Resources Conservation Service. 15 p.
Tilley, D.; Cane, J.; St. John, L.; Ogle, D.; Shaw, N. 2012. Plant guide: yellow beeplant (Cleome lutea). Aberdeen, ID: U.S. Department of Agriculture, Natural Resources Conservation Service. 3 p.
Turner, M.; Gustafson, P. 2006 Wildflowers of the Pacific Northwest. Portland OR: Timber Press. 511 p.
USDA Forest Service, Bend Seed Extractory [USDA FS BSE]. 2017. Nursery Management Information System Version 4.1.11. Local Source Report 34-Source Received. Bend, OR: U.S. Department of Agriculture, Forest Service, Bend Seed Extractory.
USDA Forest Service, Great Basin Native Plant Project [USFS GBNPP]. 2014. Seed weight table calculations made in-house. Report on file. Boise, ID: U.S. Department of Agriculture, Forest Service, Boise Aquatic Sciences Laboratory. Available: https://www.fs.fed.us/rm/boise/research/shrub/Links/Seedweights.pdf
USDA Forest Service, Lucky Peak Nursery [USFS LPN]. 2017. Seed cleaning protocols. Boise, ID: U.S. Department of Agriculture, Forest Service, Lucky Peak Nursery. Unpublished report.
USDA Forest Service, Western Wildland Environmental Threat Assessment Center [USFS WWETAC]. 2017. TRM Seed Zone Applications. Prineville, OR: U.S. Department of Agriculture, Forest Service, Western Wildland Environmental Threat Assessment Center. https://www.fs.fed.us/wwetac/threat-map/TRMSeedZoneMapper.php
USDA Natural Resources Conservation Service [USDA NRCS]. 2019. The PLANTS Database. Greensboro, NC: U.S. Department of Agriculture, Natural Resources Conservation Service, National Plant Data Team. https://plants.usda.gov/java
USDI Bureau of Land Management, Seeds of Success [USDI BLM SOS]. 2016. Bureau of Land Management technical protocol for the collection, study, and conservation of seeds from native plant species for Seeds of Success. Washington, DC: U.S. Department of the Interior, Bureau of Land Management, Plant Conservation and Restoration Program. 37 p.
USDI Bureau of Land Management, Seeds of Success [USDI BLM SOS]. 2017. Seeds of Success collection data. Washington, DC: U.S. Department of the Interior, Bureau of Land Management, Plant Conservation and Restoration Program.
USDI Environmental Protection Agency [USDI EPA]. 2017. Ecoregions. Washington, DC: U.S. Department of the Interior, Environmental Protection Agency. https://www.epa.gov/eco-research/ecoregions
USDI Geological Survey [USDI USGS]. 2017. Biodiversity Information Serving Our Nation (BISON). U.S. Geological Survey. https://bison.usgs.gov/#home
Utah Crop Improvement Association [UCIA]. 2015. How to be a seed connoisseur. Logan, UT: UCIA, Utah Department of Agriculture and Food, Utah State University and Utah State Seed Laboratory. 16 p.
Vanderpool, S.S.; Iltis, H.H. 2010. Peritoma de Candolle. In: Flora of North America Editorial Committee, ed. Flora of North America North of Mexico. Volume 7. Magnoliophyta Salicaceae to Brassicaceae. New York, NY: Oxford University Press: 205-208.
Welsh, S.L. 2016. Capparaceae A L. Jussieu Caper family. In: Welsh, S.L.; Atwood, N.D.; Goodrich, S.; Higgins, L.C., eds. 1987. A Utah flora. The Great Basin Naturalist Memoir 9. Provo, UT: Brigham Young University: 93-95.
Wyman, L.C.; Harris, S.K. 1951. The ethnobotany of the Kayenta Navaho. Albuquerque, NM: The University of New Mexico Press. p. 25.
Young, S.A.; Schrumpf, B.; Amberson, E. 2003. The Association of Official Seed Certifying Agencies (AOSCA) native plant connection. Moline, IL: AOSCA. 9 p. Available: https://seedcert.oregonstate.edu/sites/seedcert.oregonstate.edu/files/pdfs/aoscanativeplantbrochure.pdf
How to Cite
Shaw, Nancy L.; Gucker, Corey L. 2019. Yellow bee-plant (Peritoma [Cleome] lutea). In: Gucker, C.L.; Shaw, N.L., eds. Western forbs: Biology, ecology, and use in restoration. Reno, NV: Great Basin Fire Science Exchange. Online: https://westernforbs.org/species/yellow-bee-plant-peritoma-lutea/